Sustainability & the Life Sciences Industry: A Global Introduction

In this issue of Pharmaceutical Engineering®, we address an array of sustainability topics. This article surveys topics that will likely have a significant global impact on the way we conduct our business over the coming decade. We trace some history of sustainability in the life sciences industry and identify future issues of concern, including a number of areas where industry advancement cannot be made without true commitment to a full set of sustainable objectives.
The sustainability movement is now more than 50 years old; it was founded in the late 1960s and early 1970s by individuals concerned that the growing population, diminishing available natural resources, and the effects of pollution and waste could threaten the world population’s ability to survive. One notable event in this social movement was the establishment in 1988 of a United Nations commission, the Intergovernmental Panel on Climate Change, to consider the science of climate change and sustainability.
Today, the sustainability movement is growing but controversial. Recently, divergent views on climate change were evident at the January 2020 meeting of world thought leaders at the Word Economic Forum in Davos. Some attendees called for urgent action to reduce our carbon footprints; others said there is no need for concern.
For those of us in the life sciences industry, the history of the sustainability movement has overlapped with our transition into a significantly more connected, scientifically and technologically advanced era. Throughout this period, we have commenced on the Fourth Industrial Revolution, with some areas pointing to a fifth level of industrial advance. For our industry to continue to advance, we must reflect and act on sustainability concerns such as energy, waste, and water reduction.
Overview
In the timeline to reduce carbon dioxide emissions, 2020 is a significant year. The United Nations Framework Convention on Climate Change (UNFCCC) has set this year as an initial waypoint in the push toward zero carbon dioxide emissions by 2050.1 The United Nations Framework Convention on Climate Change goals have been adopted by many life sciences corporations around the world.2, 3 In nations where the drive toward carbon reduction is not as well as accepted, primarily in the US, Canada, and, to a lesser degree, Australia, the principles of sustainability are still relevant to pharma companies, which are setting goals for energy, water, and waste reduction.
The generally accepted definition of sustainability is “development that meets the needs of the present without compromising the ability of future generations to meet their own needs.”4 How this definition converts into achievable, practical goals for the life sciences industry will inevitably vary depending on the part of the world, areas of industry focus, and a company’s drivers.
Industry Response
Many multinational pharmaceutical corporations have adopted their own sets of engineering standards to ensure local compliance in a global context. These standards show a tendency of these large corporations to clearly adopt sustainable objectives.
Smaller company groups and individual companies are more likely to respond only to the expectations of their local legal and code environments unless there is a strong board or shareholder commitment or another driver, such as an ethical investor, to choose sustainable solutions. In many cases, companies may struggle to implement sustainability efforts because their facilities occupy older buildings rented or leased from third parties with little interest in sustainable objectives unless the local government forces compliance.
In the United Kingdom, efforts to meet sustainable targets are driven by planning legislation and statutory building codes that include an expectation of achieving zero carbon dioxide emissions by 2050. However, the drive toward this goal for those committed to sustainable targets is accompanied by concerns that older facilities will not be updated, given that legislation is rarely retrospective. Therefore, the onus is on individuals and companies to decide whether to adopt sustainable objectives now or delay action until laws are enforced. Notably, the latter approach can damage the public image for companies with a patient focus—a commitment to sustainability can reinforce that a company is at the forefront of promoting health and well-being.
In general, the drive toward sustainable options has been considered to be a benefit to the pharmaceutical industry, although many companies face short-term pain to achieve long-term gain. Companies often, but not always, must invest considerable amounts of money upfront to research, engineer, and implement sustainable options for processes and buildings. The results can provide large, lasting advantages for the company, such as process and operational improvements, upgraded environments, reduction of risks, and cost savings from increased efficiencies and cheaper facility operations; additionally, employees and the community can benefit from cleaner, more sustainable operations.
As companies commit to sustainability goals, they often seek out information resources and collaborative partners. Nonprofit organizations such as Science Based Targets5 and Forum for the Future6 are key players in noncompetitive, collaborative efforts on a global scale.
Science Based Targets has more than 700 corporate members worldwide, including AstraZeneca, Astellas, Biogen, Eisai, GlaxoSmithKline, Novo-Nordisk, Merck, Novartis, Pfizer, and Takeda. All have committed to zero carbon dioxide emissions by 2050.
Forum for the Future has similar sustainability commitments; its members include Walgreens, Boots Alliance, and Johnson and Johnson. The forum uses a “five capitals” model (i.e., natural, human, social, manufactured, and financial capital) to set sustainable development targets and manage improvement over time. It promotes the Net Positive Project, the Climate Futures 2030 strategy aimed at future business planning and debate, and the circular economy (which is discussed later in this article).
As Adrian La Porta, Technical Director–Process for Bryden Wood, noted in an email to the author, “Sustainability in the literature is more than carbon and water, not that these aren’t important and extremely worthwhile in their own right. The five capitals model allows you to look at financial and social impacts at the same time as environmental impacts.”
In recent years, companies aiming to manufacture and market cell and gene therapy products in the United States and advanced therapy medicinal products in the United Kingdom and European Union have opened new facilities because older facilities cannot accommodate the scientific and manufacturing requirements for these types of products. The equipment needed to manufacture these new therapies is smaller than the equipment for older product types, which means the new facilities do not need to occupy as much space. Inevitably, the stakeholders involved in designing and constructing new facilities can see the benefits of adopting leaner, cleaner sustainable standards; however, existing facilities may maintain past inefficiencies because of the difficulties of retrofitting installed clean and black utilities, outmoded HVAC systems, and other systems and equipment.
In many environments, installing LED lighting and a few solar panels on the roof is not enough for a company to claim to operate sustainably. A true commitment is much more holistic. In this respect, it is beneficial to consider sustainability strategies on the basis of product, process, and facility requirements. The coordination of these variables to improve sustainability in even a single facility can provide long-term financial gains and other benefits.
In our industry, the ways that the cost of goods (COG) and return on investment (ROI) targets are derived are important considerations for those striving to meet sustainability targets. Each pharmaceutical or biotechnology product that reaches the market requires an up-front investment in research to develop the product and rounds of clinical trials; then, the company has a 20- to 25-year patent break in which to recover costs and provide payback for the shareholders before it faces a post-patent scramble for generic supremacy in the market, during which time shareholders continue to expect return on investment.
These economic factors drive companies to set tight return on investment targets and focus on controlling the cost of goods. This makes sustainability targets tricky. From a facility perspective, return on investment/cost of goods priorities can seem to suggest that sustainability investments are “nice to have” but not cost-effective. Consequently, there is a risk that companies will lose sight of their ethical responsibilities as health providers, community members, and partners in initiatives to stop climate change. For these reasons, the success of sustainability in our industry may require national or international legislation, regulatory enforcement, or industry codes, or the strong drive and commitment of like-minded industry groups.
ISPE’s Positive Contributions
ISPE has taken a proactive role in promoting sustainability since Paul Malinowski and Nigel Lenegan established the Sustainability Community of Practice (CoP) in 2007–2008. The Community of Practice subsequently merged with the HVAC Community of Practice to form the HVAC and Sustainable Facilities Community of Practice.
ISPE has recognized and rewarded achievements in sustainability since 2009, when the Society gave the first Facilities of the Year Award (FOYA) for sustainable projects, the Facilities of the Future Award. Almost every year since then, the FOYA program has presented this award; the most recent award recipient was Celgene Corporation’s “Green Fairy Project” in Couvet, Switzerland. To see a profile of the Celgene facility and other Sustainability FOYA winners.7
The ISPE Handbook: Sustainability8 includes guidance on policy-making for sustainable objectives, along with practical suggestions for retrofit and new-build design principles and methodologies.
Pharma 4.0™, based on Industry 4.0 and the industrial Internet of Things, refers to the ongoing revolution in our industry characterized by feed-back/feed-forward data use, general improvements in in-process characterization, and advances in robotic options and equipment that are changing the face of pharmaceutical production.
Components of Pharma 4.0™ that are driving a more mature sustainable future with opportunities throughout the supply chain for improvement include:
- Advanced techniques that apply new and improved renewable materials and expand product options using additive manufacturing
- The integration of process analytical technology (PAT) in-process characterization and feedback/feed-forward data streaming to meet process integration and continuous manufacturing standards established in ICH Q13: Continuous Manufacturing of Drug Substances and Drug Products9
- Smart data applications, such as robotic process automation (RPA), that automate equipment for product manufacturing, storage, and warehousing
- Real-time particulate-level measurement and micro-metering of energy usage, which jointly provide information needed to effectively control energy use
- Improved patient-focused supply chains and drug product personalization, which minimize waste through better identification, serialization, and stock control
- System-based identification of disease outbreaks, which allows the facility to respond using modular formats and local micro-manufacturing
Collectively, these Pharma 4.0™ initiatives, along with innovations in facility and equipment design, data farming, cloud sharing, and human resources management, provide the capacity for a more focused, efficient, less wasteful, and sustainable pharmaceutical industry.
Furthermore, when Pharma 4.0™ is integrated with the circular economy concept described later in this article, this provides a new approach to manufacturing, facility design, and the supply chain as a whole. When managed correctly, this approach offers industry stakeholders the capacity to reassess existing products, their production processes, and overall supply chains and achieve significant gains in sustainability and other benefits.
In some areas, it will take time to implement such changes, and the changes may be costly at first. Companies may wonder, “If our current return on investment is acceptable, why should we invest in changes?”
QbD and Improved Processing
Quality by design (QbD), supported by ICH Q2(R2)/Q14, Q8, Q9, Q10, and Q1110, 11, 12, 13 with guidelines on analytical procedures and risk assessment, is probably the most important factor when pharma companies seek to set sensible sustainability targets from the outset of a manufacturing process. Throughout a product’s life cycle, two related issues—the technology transfer from candidate product through trials and scale-up, and, in particular, the way critical process parameters (CPPs) and critical quality attributes (CQAs) of the process are listed with the regulator—will be significant. If flexibility in the regulatory filing is unavailable, companies will be reluctant to change any aspect of formulation or method after the first regulatory clearance.
With older products, major changes to a manufacturing process usually require refiling. This is a costly exercise, which gives companies an incentive to not change the process, or to make only minimal changes so that refiling is not necessary.
Setting the correct path by responsible definition of critical process parameters and critical quality attributes ensures that companies have the opportunity to move forward to sustainable/more advanced manufacture of a new product. In some instances, they may also be able to reengineer old processes. However, the introduction of continuous manufacturing, for example, maybe challenging when older products are in constant demand and companies do not want to interrupt production. The incentives to stick with the status quo lead companies to retain inefficient, outdated, and wasteful equipment and processes, and resist changing process constituents to leaner, cleaner, and more sustainable process options that may be more beneficial over the long term.
These issues were clearly at play during a recent upgrade project, where the intention was to transfer an old solvent-based process with hydrogenation and other chemical risk issues to a new, safer, location with significant potential for process improvement and sustainable scaling of throughput. The transfer time required, the need for refiling, and the associated costs killed the project. With some process improvement through solvent-use/type re-duction, the project remains in the same unsustainable position with similar locational risk issues and little opportunity for worthwhile scaling and associated multiproduct options. “Biting the bullet” to make sustainable changes is not easy without a value proposition.
Sustainably Beneficial Risk Reduction
As indicated in the previous example, pharma industry stakeholders generally recognize that reductions in use of harmful solvents and their replacement with less-aggressive forms are beneficial and lead to easier, simpler, and safer transitions with less risk and operational cost, and without the downstream waste issues.
One tactic to reduce solvent use is to redesign elements of a process to useless. However, the better options are to look to for more beneficial methods of synthesis or to completely replace synthesis as a process with, for example, biosynthesis. Call it the “milding” of drug manufacture if the concept of “greening” is an anathema.
As Robert Dream, Managing Director of HDR Company LLC, suggested in correspondence with the author, “Let’s abandon chemicals and use a substitute, recycle what is needed and not replaceable, and use our common sense for manufacturing.”
New Products, New Approaches
As cell and gene research projects advance globally, with drug candidates transferring into clinical trials and slowly clearing their regulatory processes, stakeholders are working on feasibility studies, facility design, and site searches for new builds and retrofits suitable for these projects. Projects under-way include general-use viral vector and stem cell-based products, as well as personalized, oncology-focused products and interventions.
Given the processes and associated equipment involved, these projects are often based in small-footprint, efficient facilities, sometimes referred to as “labs+.” These facilities can handle high-throughput, small-scale processes for clinical trials batches, and, where developed to larger scale, they can offer opportunities for using continuous, smart modular processes from inception and a smoothed-out supply chain overall.
As the process scale reduces, the facility scale can also reduce, thereby providing options for more sustainable solutions with greater resource control and the potential to manufacture local to need, including in hospitals or university-based retrofits or off-site modular construction.
In the future, some new product forms may even be manufacturable through the pharma/bioequipment equivalent of a bread-making machine. Active ingredients and excipients would be directly supplied to a hospital, pharmacy, or home to suit a patient’s condition, with the machine providing the requisite dosage in a personalized form. This innovative technology would bypass the need for a facility dedicated to manufacturing, but, of course, it would still need to be fed with perfectly produced ingredients and meet rigorous standards for safe processing. Also, the sustainable benefits of such a device would need to be addressed.
Other sustainability opportunities associated with new product types may include switching to biosynthesis upstream and downstream intensification through continuous or smart batch manufacturing. These innovations would further allow reductions of scale while increasing response through process modularization combined with lean engineering techniques.
The last decade has seen the increasing acceptance and use of containment devices/isolators with better and increasing understanding of their efficient use. Drivers include the update of the European Medicines Agency EudraLex Volume 4 Annex 1,14 which is anticipated to underline the need for maintenance of high-grade backgrounds for safety cabinets and RABS devices, forcing the consideration of closed process, contained isolator–based facilities supporting lower-grade backgrounds for the surrounding volume.
This shift might eliminate the need for Grade A and B room backgrounds as processes and products are fully contained with relatively minor volumes of once-through air and the potential, subject to correct risk evaluation, for partially or fully recirculated backgrounds two grades down from the en-closed/contained volumes. This would significantly reduce energy consumption and simplify the cleanroom requirement, building response, and staff risk. Inevitably, this could provide an overall more sustainable, controlled response to the environment.
The industry may also be able to realistically consider transitioning manufacturing to clean module-based facilities that are mostly constructed off-site. This mode of construction takes advantage of the benefits of factory acceptance testing prior to site delivery and cuts down on time and resources needed to prepare and validate the site and construct the facility. These efficiencies are all sustainably beneficial.
Facility designs that aim to maximize sustainability goals allow companies to take a proactive, holistic approach to process and building assets. For example, a company could move beyond just monitoring overall energy usage to fully analyze micro-meter data on area-specific energy consumption. This more specific assessment helps target areas for improvement and can increase energy savings.
This approach to facility design, together with over 30 years of environmental assessments using tools such as Leadership in Energy and Environmental Design (LEED)15and Building Research Establishment Environmental Assessment Method (BREEAM),16 has created a new sustainability-based norm for new and retrofit facilities. However, if carbon reduction ceases to be a priority, there is a danger that company boards and engineers may query whether in-depth design assessment and investments in sustainability features are necessary in facility design.
Still, sustainability advocates can take hope that sustainability will remain a design priority. Fortunately, the business case for sustainable design can be supported by build-before-you-build principles, the use of building information modeling (BIM) design software, and other applications that let designers and engineers model real-time scenarios and time-test designs for facilities and the environments they contain before construction begins.
As a part of Pharma 4.0™, operational control in facilities is being digitalized; fewer tasks on the floor are done by humans and the worker’s primary role shifts to overseeing of digitally controlled processes within a PAT and real-time monitored space. The machine controls yield processes that are less variable due to the standardized operations inherent with automated control sequences. Such efficiencies can contribute to sustainability by reducing waste and resource consumption.
These changes to operations clearly have implications for human resources. In digitalized operations, basic human physical operational input is reduced, so fewer workers are needed. At the same time, expectations for workers increase; they must have greater skills and flexibility to run and oversee a clean, controlled facility from input to output. In sum, we can expect a transition to fewer, higher-grade staff working in smaller facilities and driving sustainable outcomes.
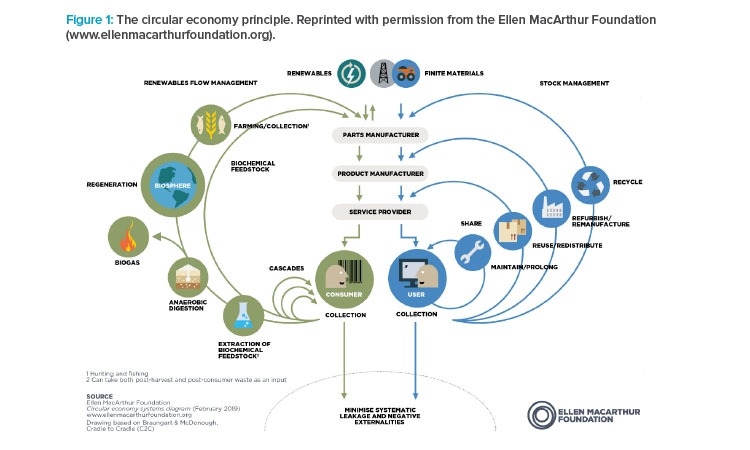
Although we have reviewed many ways that the manufacturing of drug products is becoming more sustainable, we have fewer insights about other areas such as warehousing, dispensing, and packaging. In these parts of the industry, priorities and incentives vary. For example, a primary incentive for companies to create new packaging forms is to gain advantages in the over-the-counter market; however, packaging that works well for the ROI may be resource intensive to manufacture and create large amounts of waste, which runs counter to sustainability goals.
Another area needing further consideration is the cold chain. Many drugs must be retained within the 2°C–8°C range for all or part of their processing, which is obviously consumes considerable energy. There are still many areas for sustainability-focused engineers and process architects to improve.
Further Challenges
Traditionally, economic development has been considered as a straight line that moves from raw material to product to waste. In this model, there is little acknowledgment of responsibility in sourcing or disposal. In contrast to the linear model, another model, the circular economy, has been proposed by the UK-based Ellen MacArthur Foundation and other proponents of sustainability (see Figure 1). According to the foundation’s lead statement, a circular economy “is based on the principles of designing out waste and pollution, keeping products and materials in use, and regenerating natural systems”.17
Applying the three principles of the circular economy to the life sciences industry presents a challenge that has been taken up by some significant players. Global partners in this campaign include Solvay and Unilever, and members of the Ellen A. MacArthur Foundation’s CE100 (Circular Economy 100) Network include representatives of the life sciences industry such as 3M, NovoNordisk, and DSM, as well as significant contributors to our industry such as Microsoft, Apple, Philips, Dow, and DuPont.18
Novo Nordisk and Novozymes are key partners and leaders in the Kalundborg Symbiosis, a partnership of nine public and private companies working together since 1972 to ensure that “the residue from one company becomes the resource at another,” to the benefit of both the environment and the economy.19
Adopting a circular economy approach challenges the principle of single-use plastics, where “single-use” means use and dispose, mostly through in-cineration. Such a throwaway approach in the pharma industry may reasonably be considered to be too costly once sustainability is seen as a factor, especially given the expense of the specialist medical plastics used and wider concerns about incineration as a solution to pollution.
According to a National Geographic article on hospital single-use products and the resultant waste, “In 2018, China announced it would no longer buy two-thirds of the world’s waste. That’s leaving facilities little choice but to toss their mingled plastic waste into landfills or incinerators. PVC that ends up in incinerators can release toxic chemicals”.20 According to Plastics Recycling Update, roughly 30,000 tons of biopharma single-use products are landfilled or incinerated each year.21
We cannot use plastics once and dispose of them in these ways without facing the consequences. One bright spot is that MilliporeSigma has tackled the issue directly and offers a significant service by working with their clients to eliminate waste. To quote their website, “We faced this challenge by working together with customers and the waste management industry to provide a unique process and first-of-its-kind single-stream recycling program. Our U.S.-based programs are able to recycle almost 100 percent of the products with the added benefit of traceability”.22
Conclusion
Whether by default or design, the scientific and technological advances reviewed in this article, many of which were developed over the last 10 years, provide us with an array of sustainable solutions, including:
- Changes in product formulation
- Process improvement and simplification
- Scale reduction and opportunities for continuous processing
- Data-fed automation
- Air volume reductions through use of isolation techniques
- Build-before-you-build simulation via BIM and process simulation software
Further, we can be encouraged by global agreements on strategies for sustainable solutions and the commitment of governments and organizations to timelines for major initiatives to achieve a “no waste” future and zero carbon dioxide emissions by 2050.
In the life sciences, there is the hint of a bright horizon. We were late in accepting the issues associated with sustainability, and because the ROI is uncertain, many of the feeder and front-end processes in our multifaceted industry continue to rely on old techniques that would be costly to change. We also understand that much of what is necessary to achieve successful outcomes, such as introducing a circular economy, depends on political forces that our industry can influence but can’t control. However, after a slow start, we can be proud of most of the global players in our industry for their adoption of sustainable principles. Going forward, it is important that start-ups, individual companies, and small groups participate to ensure that sustainability is a total success in the life sciences industry sector. Although sustainability issues are complex and often politicized, we can and should commit to the premise of sustainable design, construction, implementation, and operation in the pharma industry.
About the Author
Acknowledgements
Thank you to Nick Haycocks, Adrian La Porta, and Robert Dream for their suggestions about sustainability issues to cover, their constructive feedback on drafts, and their other assistance in the writing of this article.