Risk- And Science-Based Media and Buffer Mixing Validations
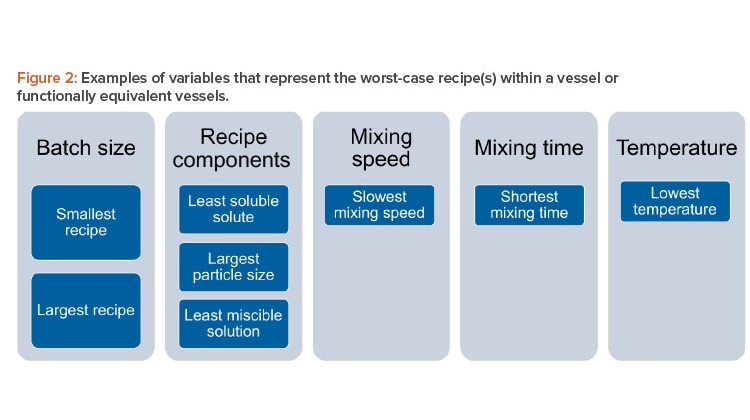
The validation of media and buffer mixing is a continuing area of resource constraint in the pharmaceutical industry. These validations require materials, validation associates’ time, and the use of equipment and processing areas. This article proposes a risk-based life cycle for minimizing mixing validation resource inputs, with the objective of optimizing validation efforts through the use of recipe bracketing and predictive methods to identify the worst-case recipes that should be validated.
Often, manufacturers expend resources to validate mixing for media and buffer recipes that are not considered the most difficult to achieve a successful mixing endpoint (henceforth referred to as worst-case recipes). Further complicating validation efforts, successful endpoint of mixing is often gauged by visual assessment of dispersion, which is subjective.
Other variables may be used, (e.g., pH, conductivity, osmolality), although they also may not be perfect indicators of solution homogeneity if the solution has not achieved turbulent mixing (often described as vortexing). Calculations and modeling are presented as viable additional tools for prediction of dispersion to leverage theoretical estimations and to prevent the repeat work associated with failed validations.
It should be noted that the presented method is a “fit most cases” approach and may not be appropriate for all programs, manufacturers, or facilities. Similar approaches may be applied to more complex mixing tasks by making additional considerations for particle sizes, densities, and higher viscosity suspensions.
Mixing in Media and Buffer Preparation
In pharmaceutical media and buffer preparation, mixing is a method by which solid substances (solutes) and liquids (solvents) are combined to create a solution with uniform concentration.
In buffer and media mixing vessels, several mechanisms work individually or in combination to create a solution of uniform concentration. These mechanisms are best described in terms of the scale at which the mechanism is occurring: macroscale, mesoscale, or microscale.
Macroscale Mixing
Macroscale mixing occurs at a scale as large as the vessel itself
the pumping action of the mixer’s impeller. The objectives of
macroscale mixing for solid–liquid mixtures are to suspend solute particles within the solvent (so that they are not sinking to the bottom or floating to the top of the vessel) and to uniformly distribute those particles within the solvent.
Mesoscale Mixing
Mesoscale mixing is the intermediate scale between the size of
the vessel itself and the microscale. At this scale, a mechanism called dispersion occurs, which is facilitated by eddy currents that create drag that involves local velocity and shear-stress effects. At this mixing scale, the objective is to distribute clumps of solid particles into smaller groups or to decrease the size of liquid droplets, creating a homogeneous size distribution of droplets.
Microscale Mixing
Microscale mixing is mixing at the smallest scale, at which viscous dissipation, molecular diffusion, and surface effects become important.
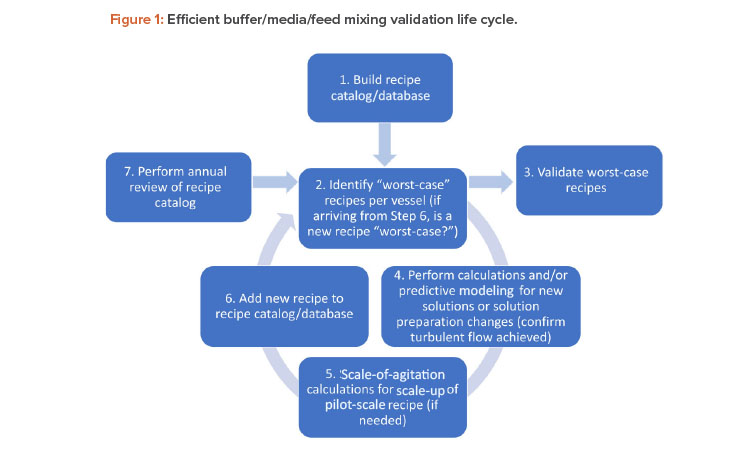
Challenges with Mixing Validation
The validation of mixing operations for all buffer, media, and feed recipes within a given organization can be a wasteful endeavor, for several reasons. First, repetitive validations are often performed. This is particularly a problem in the contract manufacturing organization space, where similar recipes are brought in by several clients, often using the same equipment configurations. Second, validations occasionally fail. Sometimes uniform mixing is not achieved with the proposed parameters and the recipe must be reformulated and revalidated.
Third, the use of an incompletely mixed solution can cause waste in the form of cost to quality due to a failed in-process control, critical process parameter, or quality attribute further down the line in the process. The quantification of successful mixing is often subjective (e.g., based on formation of a vortex or by visual judgement of dissolution) or is based on an orthogonal measurement (e.g., stabilization of pH or conductivity readings). None of these methods completely quantifies mixing at the macroscale, mesoscale, and microscale. Therefore, a small chance exists for a recipe to pass mixing validation even though the solution has not actually been uniformly mixed.
Operations and Materials in Scope
This article discusses how to determine which buffer, media, and feed recipes should be selected for physical validation of mixing during make-up within stirred stainless steel vessels and single-use mixers, and activities that should be pursued prior to performing those physical validation efforts.
Operations and Materials Out of Scope
Mixing gas into liquid and mixing a solid into another solid sometimes occurs in the pharmaceutical industry. However, these mixes are not generally used for media, feed, or buffer preparation. Therefore, those methods are out of scope for this technical article and not discussed.
Mixing within piping is important in pharmaceutical operations, and induction mixing is occasionally used for buffer and media preparation. However, the scope of this article is limited to mixing within stainless steel vessels and single-use mixers, as that is the most common method for make-up of buffers, medias, and feeds.
Other chemical and physical manipulations of media, feed, and buffer solutions during formulation (e.g., heating, sparging) are out of scope for this discussion. Only mixing at a steady temperature will be discussed in this validation bracketing/prioritization approach; this strategy may not be suited to more advanced applications.
Finally, product mixing is also out of scope for this article, as that encompasses an almost limitless material scope (subject to other process- and material-specific concerns during mixing). The design of mixing operations (e.g., use of design space) and the execution of validation activities are out of scope for this article.
Methods
This article describes ways of achieving efficiencies in mixing validation by employing a seven-step method for maintaining a risk-based mixing validation life cycle for buffer, media, and feed recipes, as shown in Figure 1.
Step 1: Build a Recipe Catalog
The first step in achieving efficiencies in an organization’s mixing validation strategy is to create an internal controlled database inclusive of all currently validated buffer, media, and feed formulations and to categorize formulations into families of which representative solutions may be validated, if required. Examples of these categorizations may include solutions with low and high concentrations, sera, low volume solutions, etc. A clear definition of each family should be established, along with criteria sufficient to sort each formulation into the correct category. This approach will make it possible to identify worst-case recipes within each family.
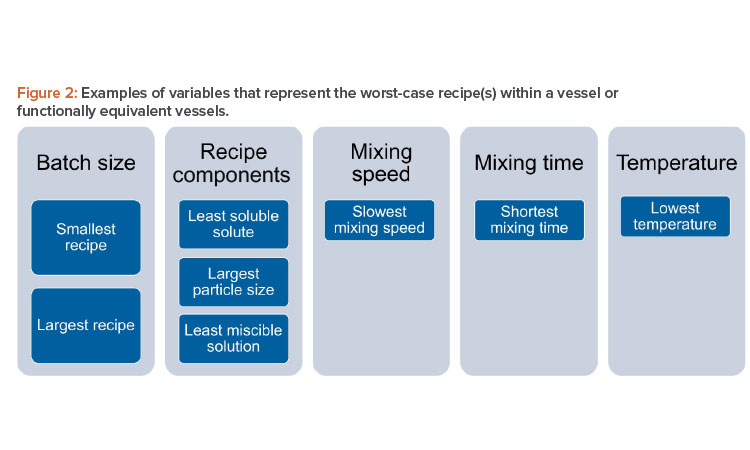
Step 2: Identify Worst-Case Recipes
This step requires identifying whether all worst-case conditions for mixing are represented within the currently validated recipes. This involves identifying what recipes represent the worst-case conditions (most difficult recipes in which to achieve successful mixing) within each vessel (and within a family of recipes).
Multiple vessels can be considered identical should they consist of the same equipment (e.g., same model/equipment ID and impeller type and dimensions) and configurational inputs (e.g., same process area, utility hookups, controller, software).
For a given vessel and impeller geometry and configuration, it is important to examine critical variables when determining which recipes that run in that vessel are worst case: e.g., batch size, solution components, mixing speed, mixing time, and temperature. Note that this list includes the most important variables, but, depending on the manufacturer’s recipe catalog, other variables may also be important.
For batch size, it will be necessary to identify the smallest and largest volumes of solution to be prepared within the vessel(s). The worst-case recipe (s) should include the recipe(s) with the least soluble solute being added within the vessel(s), the solute with the largest particle size to be added within the vessel(s), and the least miscible solution to be added to the vessel(s). The worst-case recipe(s) should also include the recipe(s) with the slowest mixing speed, shortest mixing time, and lowest mixing temperature. Figure 2 depicts a summary of this approach. It includes critical variables that can inform a family approach/grouping of recipes.
Weighting may be applied to the variables indicated to demonstrate relative importance to product quality, e.g., formatting of the database as a custom cause-and-effect matrix or other similar criticality assessment tool. In the interest of efficiency, the current validation strategy should evaluate the fewest recipes possible that represent the worst case for critical variables within a given vessel geometry and configuration (i.e., solution family).
It should be noted that the structure of recipes matrix (database) will vary significantly for each manufacturer and will depend on recipe media/buffer family types, groupings of equivalent equipment, and variables chosen due to specifics of the recipes within a given family. Variables to be considered for grouping should include variables in Figure 2 as a starting point and then possibly include additional variables dependent on the recipe characteristics.
A cross-functional team should approve the finalized database, including experts from engineering, manufacturing sciences, quality assurance, validation, risk management, and manufacturing. However, the recipes evaluated must represent actual formulations for the process(es) being validated.
Step 3: Validate Worst-Case Recipes
If review of the recipe database revealed that some worst-case media, buffer, or feed recipes were not previously validated for a given vessel configuration, then mixing of those recipes should be validated.
Step 4: Confirm Turbulent Mixing Will Be Achieved
This step requires performing calculations and/or predictive modeling for new solutions, or when a solution preparation process changes, to evaluate whether turbulent mixing is going to be achieved. When changes to equipment, batch size, ingredients composition, etc. occur, affected recipe(s) should be subject to calculations or modeling that predict whether turbulent mixing will be achieved.
Dimensionless number (e.g., Reynolds number) calculations can be applied as previously described
Figure 3: Reynolds number formula.
\( N_{Rc}= \frac{D^2pN}{ \mu} \)
where
N = shaft speed (sec-1)
D = Propeller blade diameter (cm)
p = density of solution dispersion (g/cm3)
\(\mu \) = viscosity of solution dispersion (g/[cm/sec])
Variables Known | Variables to Be Determined | Necessary Assumption |
---|---|---|
|
| The mixing time of the pilot and scaled-up batch is the same |
It should be noted that a relationship exists between pumping number and Reynolds number within the turbulent range that is close to a straight line.
Performing these dimensionless number calculations in advance of starting mixing validation work will save effort overall because, if validation fails, the recipe design will need to be reevaluated. If lack of turbulent mixing is detected via dimensionless number calculations (prior to mixing validation), resources (e.g., equipment, suite time on the schedule, personnel hours, materials, etc.) will not have been wasted.
The authors have observed in the last few years a rise in the use of computer simulations and artificial intelligence applications that evaluate the mixing of solutions. This further strengthens the authors’ message that these simulations are very useful. For example, numerous studies have been recently performed on fluid mixing simulation via computational fluid dynamics platforms validated for a specific equipment configuration in stirred tanks and bioreactors.
Modern software platforms can identify mixing dead zones within a vessel
Step 5: Calculations for Scale-Up of Pilot-Scale Recipes
If scale-up of a new recipe is desired, once turbulent mixing of the pilot-scale recipe has been confirmed via dimensionless number calculations and/or a validated computational model, the scale-of-agitation approach can be used to determine scale-up batch parameters.
If the facility has a validated predictive modeling application in place, this again can also be applied to further confirm predicted mixing success of the scaled-up recipe.
Step 6: Add the New Recipe to the Database
Having received this confirmation that a new recipe is expected to achieve thorough mixing, the next step is to add the recipe to the database. Then, proceed back to step 2 to determine whether the new recipe is within the current recipe catalog/database bracketing design or represents a new worst-case recipe.
To understand whether a new recipe is represented within the existing bracketing strategy (or, alternatively, whether that recipe represents a new worst case that should be added to the current bracketing strategy), the following questions should be addressed. This will help identify if this recipe represents a new boundary condition for any of the critical variables previously discussed within the vessel(s) where it will be prepared:
- Does this new recipe represent a new smallest/largest volume of solution to be prepared within the(se) preparation vessel(s)?
- Is a solute in the recipe less soluble than the one that was previously considered as worst case for the(se) preparation vessel(s)?
- Does the new recipe contain a larger particle size than was previously evaluated in the(se) preparation vessel(s)?
- Is a new solution component less miscible than solution component(s) that were previously evaluated as worst case in the(se) vessel(s)?
- Is this recipe’s mixing speed/time and/or temperature lower than those which were previously evaluated as worst case for the(se) vessel(s)?
If the answer is yes for any of these variables (or if the weight score is the new highest within a solution category), this recipe represents a new worst case and should be validated (proceed to step 3).
Step 7: Review Annually
It is recommended that the database or matrix of buffer, media, and feed recipes should be evaluated periodically based on a science- and risk-based rationale. This review period should not be longer than three years, according to existing regulatory expectations for validation of other systems (e.g., controlled temperature units).
Conclusion
Efficiencies in mixing validation can be achieved by application of a life cycle approach. By developing a catalog of media, buffer, and feed recipes, it is possible to employ a bracketing approach in which only the worst-case recipes within a given equipment configuration are evaluated. New recipes or changes to existing recipes can be verified for turbulent mixing with calculations and/or predictive modeling prior to the occurrence of any physical mixing validation.
Where scale-up of a new recipe is needed, one may employ a scale-of-agitation calculation approach to ensure the mixing conditions from the smaller (pilot) batch are preserved. New or changed recipes can then be assessed to determine whether they represent a new worst case that must be validated for a given vessel or functionally equivalent vessels.