2020 ISPE Barrier Survey: Tracking the Journey of Barrier Technology
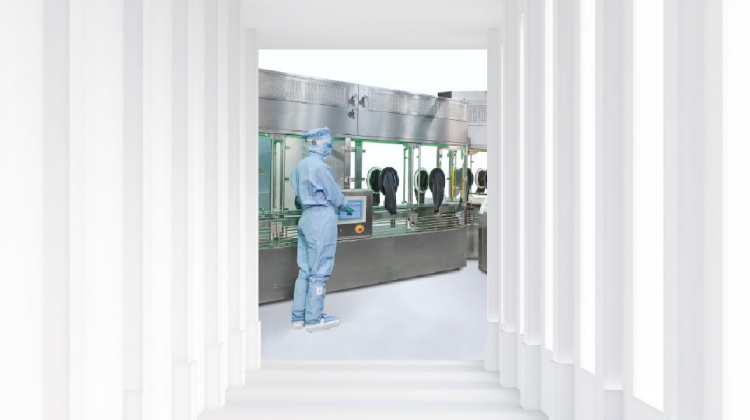
For over two decades, the ISPE Barrier Isolator Survey has gathered meaningful data on the applications of barrier technology and been a resource for the fill-finish pharmaceutical industry community. This article provides context for the latest survey, the first in several years, and presents its key results, which were first shared at the 2020 ISPE Aseptic Conference in North Bethesda, Maryland, in March 2020.
Unit operations involving aseptic processing are critical to product integrity and safety in the drug manufacturing process. The cleanliness of the environment surrounding these processes and adherence to associated standard operating procedures ensure that the drug delivered to the patient is sterile and essentially free of particulates. Aseptic processes include the filling of injectable products into final containers (in the form of vials, syringes, cartridges, ampules, and others) and aseptic formulation of suspensions or live virus vaccines that do not undergo subsequent sterile filtration. Given the current increase in other small-scale aseptic applications, including cell and gene therapy processes and 503A and 503B compounding pharmacies, aseptic barrier technology is needed now more than ever before.
Origins of Aseptic Barrier Technology
Those new to the world of aseptic processing may take the now mature solutions of today’s robust barrier isolators and restricted access barrier systems (RABS) for granted. However, it is important to note the challenges the industry faced before arriving at the current state of barrier technology. A mix of innovation, failure, debate, improvement, and persuasion was required to overcome the obstacles impeding broad implementation.
Through the 1980s, aseptic processing for formulation and filling operations for injectable products was performed solely in traditional Grade A cleanrooms. Product protection in traditional aseptic processing relied exclusively on the unidirectional flow of HEPA-filtered air combined with good aseptic technique by fully gowned operators. Activity by operators in proximity to open processes sometimes led to product contamination.
When environmental monitoring revealed that operators were often the greatest source of process contamination, the concept of barriers emerged, starting in the form of machine guards, curtains, and other devices intended to separate people from processes. These early barriers still relied heavily on aseptic technique for nonroutine activities as well as many routine operational tasks. Thus, the initial attempts to enhance aseptic processing by implementing flexible curtains or simple guarding represented small, but inadequate, steps forward. Processes and equipment still frequently relied on operators to bypass the barriers. There had to be a better way.
By the late 1980s, barrier isolation technology for fill-finish processing was under development. It was thought this new technology could take the human factor out of this critical process step. However, obtaining approval from regulatory agencies for this novel approach could be difficult.
Trailblazing efforts involved struggles with defining appropriate and feasible levels of decontamination—i.e., sterilization versus biodecontamination. Hydrogen peroxide vapor became the chemical agent of choice; however, in the early years of this technology, biodecontamination cycles were very long, with many lasting over 10 hours. News of the initial limitations, high cost, and challenging qualification efforts of barrier isolators led many to doubt the practicality and efficacy of the technology.
In parallel to the long-term development of barrier isolators, industry professionals recognized the urgent need to improve barriers for aseptic pro-cessing beyond curtains and simple guards. Without an isolator, how could operators be separated from the process during routine processing tasks and even during process interventions? Iterative design improvements enhanced simple machine guarding with glove ports, material transfer solutions, active air handling, and other innovations. With these improvements, a new name for a truly engineered solution was warranted: restricted access barrier system (RABS).
In the early 1990s, industry leaders worked within ISPE to establish a new platform for end users to discuss isolators and advocate for their adoption, which resulted in the formation of the ISPE Barrier Isolator Conference (now the ISPE Aseptic Conference). At this conference, the idea for a new industry group was formed: the Barrier Users Group Symposium (BUGS).2, 3, 4 This group—made up of representatives from Merck, Upjohn, Eli Lilly, Bayer, Sanofi, TL Systems, Despatch Industries, and the University of Minnesota—would lead the way in the adoption of barrier isolator technology in their final drug-filling operations.
To help ensure the US FDA would support barrier isolator adoption, a small subgroup emerged—LUMS. The companies involved in the LUMS sub-group (Lilly, Upjohn, Merck, TL Systems, Despatch Industries, and the University of Minnesota) worked together to build a model isolator system and generate data with media-filled containers.2, 3, 4 In 1995, these media fill data were shared with the FDA and served as evidence that isolator technology could be used to significantly improve the cleanliness of the area immediately surrounding the drug final container closure (vial). Isolator technology increased drug safety for the patient while also reducing operating costs.
Barrier isolator technology has advanced significantly since then. Better air handling systems, shortened decontamination cycle times, and more complete validation packages are just a few of the notable advancements.
Survey History
Tracking the number of installed barrier/isolator systems and the evolution of barrier technology is important to end users and equipment manufacturers alike. Understanding this progression can help ensure the best technologies are considered and incorporated into all new fill-finish projects. Data about the application of the technology can also highlight gaps and areas for improvement.
The ISPE Barrier Isolator Survey, which was first established in 1998 by Jack Lysfjord and Michael Porter, has shown the trends of increasing adoption of isolator and restricted access barrier systems installations and evolution of the associated technologies. For 15 years, Lysfjord and Porter tracked various metrics and reported survey results at the ISPE Aseptic Conference on a biannual basis: isolator survey results were reported for 1998, 2000, 2002, 2004, 2006, 2008, 2010, and 2012; and RABS survey results were reported for 2005, 2007, 2009, and 2011.5
The survey results provided data on the progression of technology adoption and documented many technological and operational improvements related to the design of isolator and restricted access barrier systems. The current survey, now called the ISPE Barrier Survey, researched all barriers including isolators and RABS.
Definitions
As the design attributes of isolator and RABS systems took form, it became possible, and necessary, to refine their definitions to enable broader implementation. Understanding the key attributes of RABS and isolators, and the distinctions between the two, enables well-informed technology selection as well as interpretation of the survey data presented in this article.
RABS
An example of one of the first RABS applications in the early 1990s illustrates their role in separating people from the process for tasks that previously relied solely on aseptic technique by gowned operators.
The example operation involved collecting sterile stoppers at the discharge of a stopper processor. The discharge was located fairly low to the floor, causing the operator to lean over the discharge and block the “first air” as the stoppers were accumulated. The “first air” principle in aseptic processing is that no object shall be positioned in the unidirectional flow airstream between the HEPA filter and the critical process zone, thus ensuring that the critical process is exposed to “first air."6 Innovative designers provided a combination of guarding and strategic access points to keep the operator segregated from the discharge point and away from the first air while still installing a receiver chamber under the stopper processor. According to the story, when asked what this device was called, the originators said it was a restricted access barrier system, and RABS was born.
The example of the stopper processor was a step in the right direction, and the concept of RABS has progressed much further since then. The definition for RABS in the recently published draft of Annex 1 7 is as follows:
System that provides an enclosed, but not sealed, environment meeting defined cleanroom conditions (for aseptic processing Grade A, but where used for non-sterile applications can be lesser grade) and using a rigid-wall enclosure and air overspill to separate its interior from the surrounding environment. The inner surfaces of RABS are disinfected and decontaminated with a sporicidal agent. Operators use gloves, half suits, rapid transfer systems (RTPs) and other integrated transfer ports to perform manipulations or convey materials to the interior of the RABS. Depending on the design, doors are rarely or never opened.
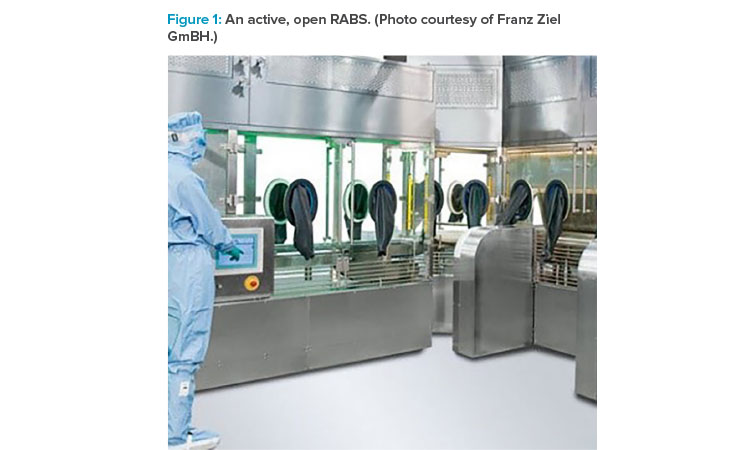
A well-executed RABS design emphasizes the system aspect—i.e., the RABS is an integrated and enabling part of the overall aseptic process. A RABS is not just rigid machine guarding that is opened during operation. Instead, it is a system that also includes glove ports, often rapid transfer ports (RTPs), and other features to provide operator segregation at all times and enable uninterrupted aseptic processing.
Risk assessment during RABS design determines required features and procedures that must be in place to permit nonroutine interventions. The system (machine and procedures) must enable intervention alerts and tracking, product tracking, and determination of product rejection or batch loss for various types of interventions.
The Annex 1 draft classifies RABS as opened or closed. The term “open RABS” can be misunderstood to mean that the doors of the barrier are rou-tinely opened during operation. In fact, the word “open” refers to the way first air from the HEPA filter flows over the process and then out into the room. In an open RABS, the air typically exits the barrier through slots or grates at the bottom of the barrier. Figure 1 illustrates that the system is open in the way air flows downward from the HEPA filters through the barrier and out to the room through openings below the level of open containers. The doors remain closed during operation.
Two subsets of open RABS are active RABS and passive RABS, which are differentiated by the HEPA air supply sourcing. An active RABS includes fan fil-ter unit(s), with fan and HEPA filters integrally mounted to the barrier frame (see Figure 2). The barrier system in a passive RABS uses the room HEPA filtration and first air supply. In a passive RABS, the top of the barrier is positioned closely enough to the HEPA supply to capture and channel the flow past the process (see Figure 3).
![Figure 2: Active RABS with onboard air management [8].](/sites/default/files/2020-06/0720_PE_JA_CoverStory_03_0.jpg)
![Figure 3: Passive RABS using unidirectional airfl ow coverage from the cleanroom [8].](/sites/default/files/2020-06/0720_PE_JA_CoverStory_04_0.jpg)
![Figure 4: Example of a closed isolator. (Reprinted with permission of VanRx; photo of Emergent BioSolutions closed robotic workcell [gloveless isolator].)](/sites/default/files/2020-06/0720_PE_JA_CoverStory_05%20copy_0.jpg)
Isolators
The 2020 Draft of the Annex 1 offers the following definition for isolator:
A decontaminated unit, with an internal work zone meeting Grade A conditions that provides uncompromised, continuous isolation of its interior from the external environment (e.g. surrounding cleanroom air and personnel).
Annex 1 further identifies two types of isolators:
- “Closed isolator systems exclude external contamination of the isolator’s interior by accomplishing material transfer via aseptic connection to auxiliary equipment, rather than use of openings to the surrounding environment. Closed systems remain sealed throughout operations.” (See Figure 4.)
- “Open isolator systems are designed to allow for the continuous or semi-continuous ingress and/or egress of materials during operations through one or more openings. Openings are engineered (e.g. using continuous overpressure) to exclude the entry of external contaminant into the isolator.” (See Figure 5.)
Each type of isolator has distinct advantages and specific uses. Closed isolators tend to be highly used for lower-speed, lower-
volume applications, and where containment is a concern. They are operated in a batch operational scheme, and all materials for the aseptic process are either placed inside the isolator prior to biodecontamination or introduced afterward by a closed transfer process, such as a rapid transfer port or H2O2 airlock.
Open isolators allow higher-speed applications and continuous operational schemes, and can use air pressurization bubbles and sinks to accommodate containment. They typically involve integrated systems such as a vial-filling machine that receives components from a depyrogenation tunnel via a low-profile gate and discharges them to a capping/sealing machine via a small-profile “mousehole.” Although the isolator is open to the tunnel and cap-per, proper pressurization and local HEPA coverage at the boundary openings ensure protection of the aseptic environment.
As noted previously, isolators have gained significant acceptance within the industry. Complete segregation of the process from operators and the surrounding environment, coupled with automated and validated biodecontamination processes, elevates them as the barrier method most likely to meet regulatory expectations. During FDA panel discussions at the conclusion of recent ISPE Aseptic Conferences, FDA representatives have repeatedly indicated that they expect facilities equipped with a minimum of RABS to receive less scrutiny than facilities with traditional aseptic processing setups. They said that facilities equipped with isolators are viewed most favorably.
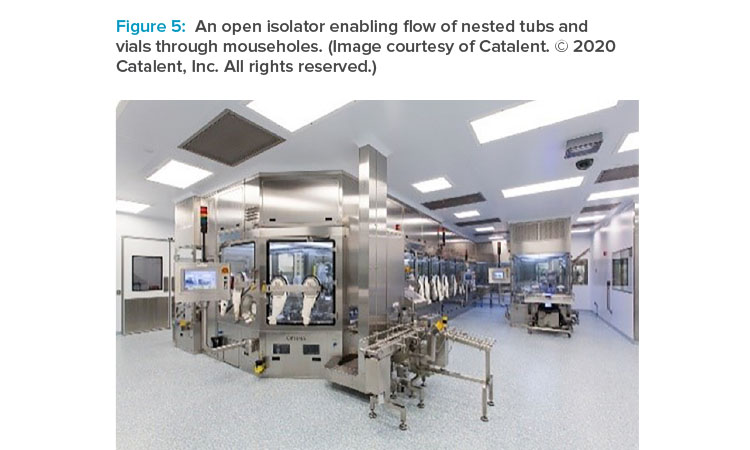
New Survey Design
Understanding the trends in both RABS and isolator designs and installations can help guide engineers working on current fill-finish projects in both old and new facilities, and help equipment manufacturers better understand market needs. Although the last survey by Lysfjord and Porter was conducted in 2012, interest in related trends continued. In 2019, a work group formed within ISPE to revive the survey and collect data from the past five years, with the intent to fill the gap and publish data on the equipment installations that occurred since 2012. The group had big shoes to fill, so its membership was composed of several industry peers with decades of experience in aseptic processing.
The current ISPE Barrier Survey group wanted the information they gathered to be as useful, accurate, and complete as possible. The group first met in May 2019 to discuss the objectives for the new survey and capture their goals in the following mission statement:
To develop a thought-provoking survey on the implementation of RABS and isolator-based systems across a range of manufacturing scales in the pharmaceutical industry which contains relevant information to convey important trends and data to the ISPE community.
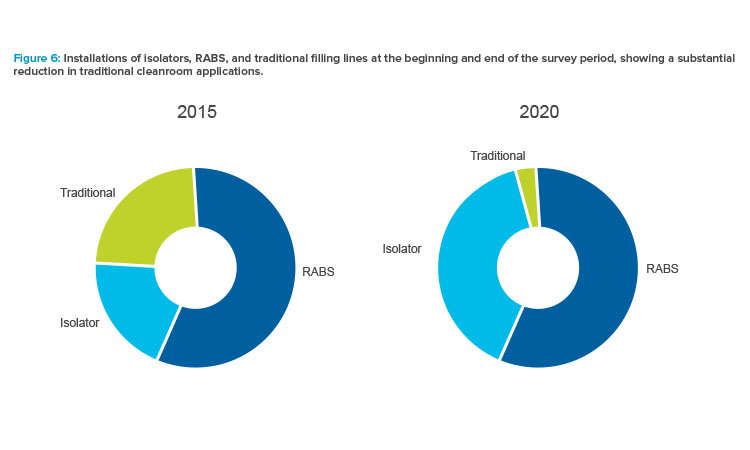
The group determined the best way to collect meaningful data would be to approach the equipment manufacturers directly, which would ensure that the collected data were representative of a broad range of applications. The group reached out to original equipment manufacturers (OEMs) known for supplying major markets across the globe. They assured the companies that data would remain confidential and would only be used to show trends, and the response from original equipment manufacturers was very positive.
Once the survey group had buy-in from the original equipment manufacturer community, they compiled the survey questions, which asked for specific details related to the filling equipment, associated barriers, containers being filled, installation locations, and more. The group requested that survey participants provide these details for every individual project since 2015. Survey details and responses were captured and organized for analysis using a spreadsheet in late 2019. Even though this was a huge ask of the 13 participating suppliers, the response rate of over 90% was good, and data were collected on over 900 global installations.
Survey Results
The results, representing over 900 filling applications from the participating industry suppliers, showed some interesting and welcome trends. The most relevant and intriguing results are outlined here. The presentation from the 2020 ISPE Aseptic Conference with all results has been posted on the ISPE Sterile Products Processing Community of Practice site and on the website for the 2020 ISPE Aseptic Conference attendees.
The most notable outcome was the clear trend toward the use of barrier systems and the near extinction of “traditional” cleanroom installations for aseptic filling (see Figure 6). Respondents indicated that, in 2020, virtually no systems would be delivered without a form of RABS or isolator, illustrating that the industry is decidedly moving to the use of barrier systems. This is great news for patients and product safety.
Reviewing the results, some may wonder why isolators were not used in a larger proportion of projects. The authors recognize that a contributing factor could be the common practice of retrofitting existing facilities, which frequently have Grade B infrastructures and height limitations. These projects tend to use RABS as the more economical and easily constructed alternative. Attributes of the product being filled and its associated manufacturing processes also impact the choice of RABS or isolator. Many factors should be considered when evaluating which barrier type is most appropriate to use for a project.
Trends related to fill speeds were also studied, and the data represented a wide range of unique fill speeds. Results were grouped into four general categories based on units of production per minute: less than 50, 50–199, 200–400, and greater than 400. These groupings were chosen to reflect representative industry offerings and applications. Between 2015 and early 2020, the proportion of equipment supplied by line speed remained fairly constant. Most of the applications fell in the 200–400 units per minute range. Figure 7 shows that the North American market had a higher percentage of low-speed lines than Europe and Asia, and high-speed filling lines made up the majority of lines in the Asia market. The number of projects reported in the survey for Africa and South America were too few to include the graphing of region-related results.
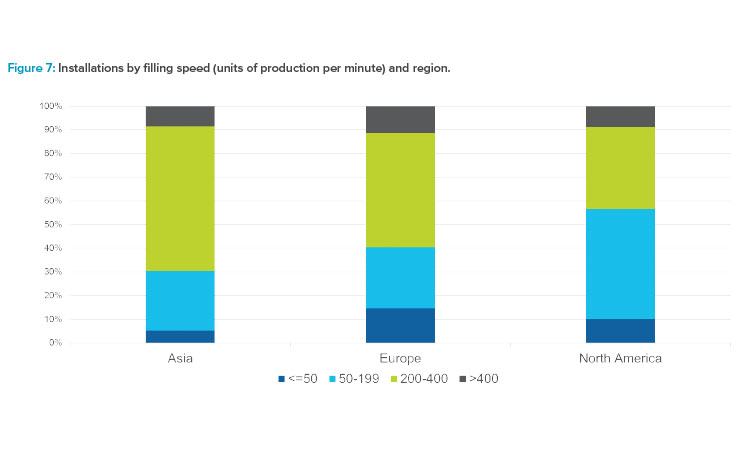
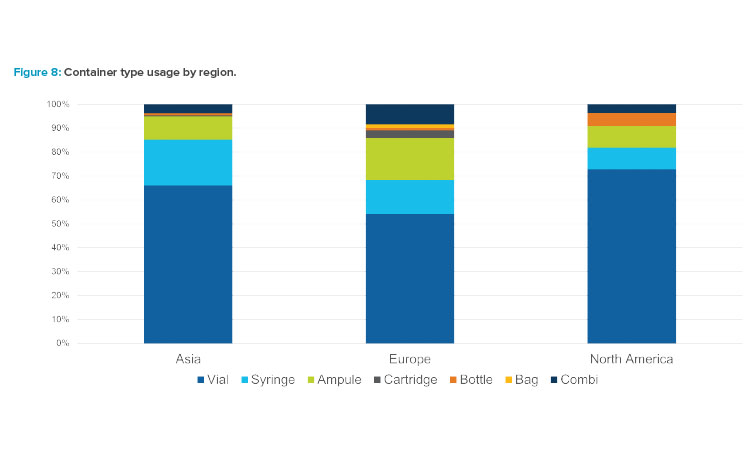
The survey also evaluated final container type. Figure 8 illustrates that vials represented the majority of container types, with proportions varying slightly by region. In each region, syringes and ampules were used in about 10%–20% of the installations. Compared to the other regions, Europe installed a larger percentage of ampule fillers during the survey period. Although combination/flexible fillers, which are capable of processing more than one component type, are often discussed these days, the survey data did not include a significant number of combination filler projects.
Figure 9 shows the types of barriers used for different container types. Barrier technology was most often used for syringe applications, and isolators were used for a higher percentage of these applications compared to other formats. Syringe lines predominantly use presterilized, nested-format components in tubs that are contained in sealed bags. Use of isolators is ideal for these setups because isolators enable the tubs to be debagged in one place, with the barrier technology keeping people out of the process and simplifying the transitions from Grade C to Grade B and from Grade B to Grade A.
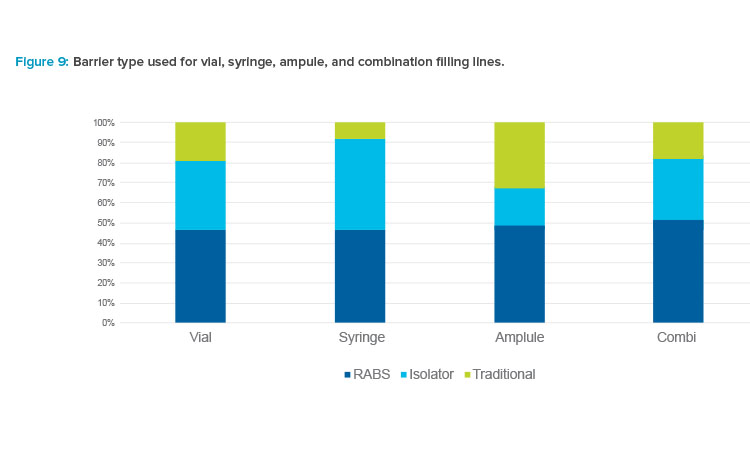
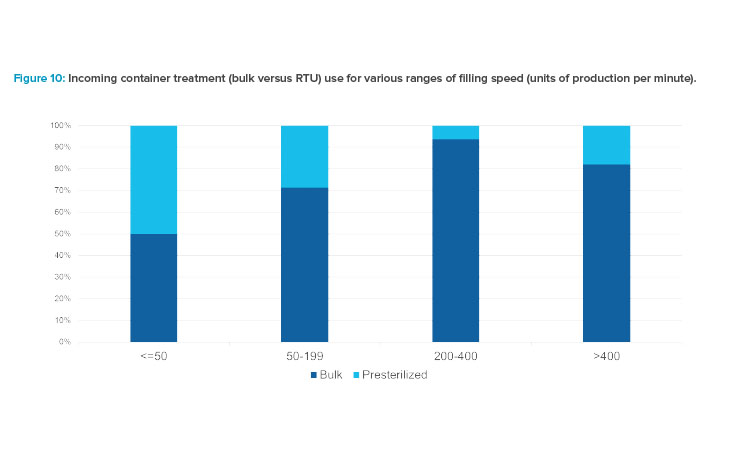
Survey results indicated an interesting relationship between filling speed and the type of container supply format. Figure 10 compares the percent-ages of incoming container types—bulk containers (vials requiring use of vial washer and depyrogenation tunnel) versus ready-to-use (RTU), or presteri-lized, containers—for the four ranges of filling speed.
It is helpful to review several factors that influence the choice of bulk or presterilized components and also the type of barrier in which they are processed. Presterilized components cost more per unit compared to bulk components. Costs of either type vary largely by volume purchased annually. Bulk components run through a washer and depyrogenation tunnel prior to entering the filling line. Presterilized components are introduced by a con-trolled debagging, no-touch transfer process or through a decontamination process, such as e-beam or biodecontamination. Nearly all syringe filling applications use presterilized units, supplied in nested format. In evaluations for the choice of bulk versus presterilized components, cost implications for components, equipment, labor, utility, infrastructure, and material handling should be considered.
Considering the points above, it is not surprising to see that the highest usage of presterilized components occurs in the two slower speed ranges. Lower-speed filling lines with smaller batches and, typically, higher-value products are more often coupled with
presterilized components. Bulk components were most prevalent in the 200–400 units per minute range. The increase in usage of presterilized components for the fastest range is certainly represented by filling of presterilized syringes, as it is not cost-effective or practical to run at high speeds with presterilized vials. Data also indicated that the use of bulk supplied vials remained fairly constant over the survey period, at about 80%–90% of the applications overall.
Figure 11 illustrates the tendency for production using presterilized containers to be installed in barrier systems. In comparison, traditional cleanroom installations were used for more than a quarter of for bulk component feed applications.
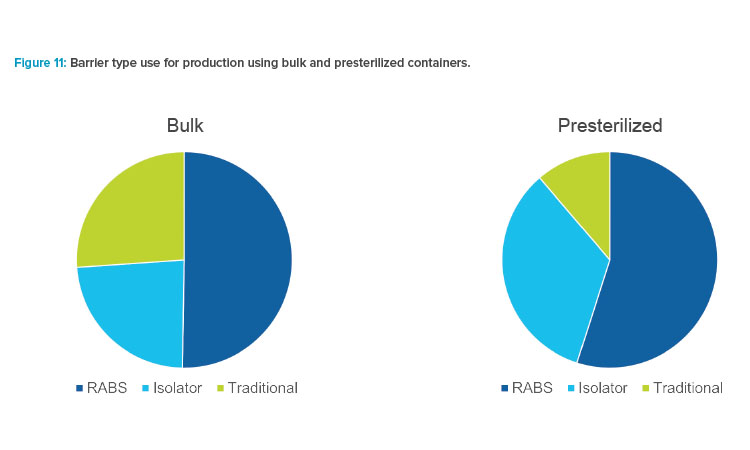
Results for terminally sterilized products were limited, but the available data indicated a much higher usage of RABS over isolators for terminally sterilized products. Due to different regulatory requirements for manufacturing terminally sterilized products, many manufacturers choose to operate with less-stringent microbial controls since the final process step assures product sterility.
Lyophilized products represented a small portion of the results. The data showed use of lyophilization for product preservation held steady at 20% of the applications over the survey period.
Conclusions
Taking part in the renewal of the Barrier Survey has been a privilege for the ISPE Barrier Survey team. The results of the survey indicate continually in-creasing application of barrier technology for advanced aseptic processing since 2015. Interrelationships between barrier type, line speed, region, container type, container processing, and other categories help us understand the current landscape of sterile drug product manufacturing.
Perhaps most notably, the renewed survey may have captured the approaching end of traditional cleanroom processing for new aseptic applications. Its data help us recognize how far the industry has come since the efforts of early implementers of barrier technology. This has been a journey with high hopes and some setbacks. The trailblazers in barrier technology development might be pleased with some of the survey results—and yet they would likely yearn for more, such as broader implementation of isolators, increased acceptance of data-driven strategies, and new methods for environmental monitoring. The survey team and countless industry colleagues also aspire for more.
Progress continues. Years ago, the most successful solution providers made a powerful transition, leaving behind their identity as machine builders to become innovation leaders. A select few suppliers initially gained the know-how to advance methods and offer robust solutions. Fortunately, the number of qualified suppliers continues to grow, allowing the global machine-building capacity and number of isolator installations to increase.
Progress often leaves someone behind. The impressive recent advancement in barrier technology sharpens the differences between new installations and older manufacturing technology in legacy facilities. Regulatory inspectors may observe a state-of-the-art isolator installation one week and a decades-old facility with a traditional Grade A cleanroom the next. Presenters on the FDA panel at the 2020 ISPE Aseptic Barrier Conference confirmed that legacy facilities receive a higher level of scrutiny because the patient safety risks are inherently greater for traditional aseptic processing than for aseptic processing using barrier technology.
When some drug manufacturers implement new and improved capabilities, others feel pressured to maintain pace. Fortunately, a leapfrog opportunity is at hand for companies in need of modernizing their aseptic processes. Although the regulatory bar continues to rise, the hurdles for entering the world of advanced aseptic technology have been lowered. Thanks to work by predecessors, organizations can take advantage of budget-friendly, state-of-the-art technologies. New filling lines offer speed, accuracy, flexibility, and reliability. Isolator solutions come with well-developed H2O2 decontamination technology and glove-testing solutions, and because costs are lower, more drug manufacturers can afford to invest in barrier isolators. In addition, new compact designs enable the installation of lines in smaller spaces, including opportunities for cost-effective facility renovations.
During discussion after the presentation of the survey results at the ISPE 2020 Aseptic Conference, one attendee exclaimed, “Mission accomplished!” Indeed, the industry has achieved broad implementation of barrier technology and related process improvements. However, those passionate about aseptic processing still see great opportunity for continuous improvement. Here’s to the next 30 years of innovation.
Acknowledgments
The current ISPE Barrier Survey group is composed of Jessica Frantz, lead, (Sartorius); Lisa Dorn (CRB); Charlotte Enghave Fruergaard (NNE); Jorge Ferreira (Jacobs); Christa Myers (CRB); and Paul Valerio (IPS).
Appreciation goes to the suppliers contributing data for the survey, including Airex, Bausch+Stroebel, Bausch Advanced Technologies, Comecer, Dara, Franz Ziel, Groninger, OPTIMA Pharma, Shibuya, Skan, Steriline, Syntegon, and Telstar. Special thanks go to Jack Lysfjord and Michael Porter for their role in spreading the message in earlier days, as well as many others whose efforts led to much progress over the years.