Quality By Redesign of a Legacy Product Case Study

In recent years, the concept of quality in the pharmaceutical industry has evolved from the idea of testing the quality to designing the quality. The fundamental idea is very simple; it is necessary to understand the material and process variables that determine the final product’s quality from the beginning of product development. Such an approach permits an in-depth understanding of the product and guarantees its quality by adjusting process variables based on known variability of input materials and intermediate manufacturing phases. The strategic thinking is based on the fact that processes might change or drift over time, which gave birth to the well-known quality by design (QbD) approach.1
- 1PQLI Roadmap: Product Design, Development, and Realization: A Science-and-Risk-Based Approach to Implementation. PQLI Guide Series, ISPE. 2010.
Background
The concept of QbD has been successfully implemented in many industries for decades. However, it required time before it could be implemented in the pharmaceutical field, mainly due to the lack of regulatory harmonization among different countries and regions. To ease the implementation of QbD, and generally harmonize the quality concept, ICH released several guidelines, ICH Q8–Q11,2 , 3 , 4 , 5 which provide a general framework for QbD application to drug substance and drug product science-and risk-based development and manufacture.
Case Study Review
Since the release of ICH guidelines, several case studies were published on how to apply the key elements of QbD to product development for both chemical and biological/biotechnological entities.6 , 7 ,8 ,9 Furthermore, in collaboration with the Pharmaceutical Control Services of the Health Department of the Catalan Government, the ISPE Spain Affiliate published a case study about the application of QbD to legacy products.10 In this article, a case study of QbD applied to a lyophilized injectable drug product is presented. The product has been already marketed by Laboratorio Reig Jofre since 2008 as a generic version of a reference drug product in different markets, including Europe (EU), Israel, South Africa, Hong Kong, Vietnam, and Georgia.
The introduction of a new larger freeze-dryer, which drove an increase in batch size to match the new machine’s full capacity, inspired this project. At the same time, it was determined useful to review knowledge gained during initial development and routine manufacturing (approximately 3–5 batches per week for 8 years), and propose improvements where necessary, applying a risk-based approach throughout the process. This would generate the first set of variations, compared to the already-approved dossiers. Furthermore, at the same manufacturing site, a new manufacturing zone with increased capacity for sterile injectables was to be constructed, with plans to transfer the product to this new manufacturing area, with larger freeze-dryers. This will represent the second group of future variations.
Variation classification depends on each country’s regulation, but in most of the cases, at least several major variations will be necessary. In Europe, these changes are classified as Type II variations (also taking into account that freeze-drying is considered a nonstandard manufacturing process). Approval and implementation of such changes usually require up to 2 years. To reduce this time, and make the product manufactured with the new process and/or in the new facility commercially available sooner, an additional variation can be filed to include a Post-approval Change Management Protocol (PACMP), according to the current European legislation. This document lists the foreseen changes as well as proposes a strategy for evaluating and mitigating potential impact on product quality.
Aemps Collaboration
If supporting data and strategy are sufficiently sound, from both a scientific and a risk management point of view, it is possible the regulatory authorities will downgrade the variation type—in this case from Type II to Type IB, or even IA. If approved, this permits for faster evaluation of the proposed changes with a consequently shorter time for commercial implementation. Although appealing, this approach is not commonly used to introduce future variations. Considering the complexity of the aforementioned changes, it was decided to contact the Spanish national health authority Agencia Española de Medicamentos y Productos Sanitarios (AEMPS) and share with them the details of the project plan and proposed strategy.
The initiative was well accepted by the AEMPS. In an initial meeting, a QbD approach was proposed for the revision and redevelopment of the product. It was accepted that the scope of the subsequent meetings would be the first group of variations, i.e., QbD-based variations and the introduction of a PACMP. At the same meeting, Laboratorio Reig Jofre proposed a working strategy that included several meetings between Laboratorio Reig Jofre and AEMPS to review previous project milestone outcomes and coevaluation of the strategy to be implemented for subsequent milestones. Figure 1 provides a schematic representation of the milestones and proposed meetings.
- 2ICH Harmonised Tripartite Guideline.Q8: Pharmaceutical Development. ICH, 2009.
- 3ICH Harmonised Tripartite Guideline.Q9: Quality Risk Management. ICH, 2011.
- 4 ICH Harmonised Tripartite Guideline.Q10: Pharmaceutical Quality System. ICH, 2008.
- 5ICH Harmonised Tripartite Guideline. Q11: Development and Manufacture of Drug Substances (Chemical Entities and Biotechnological/Biological Entities). ICH, 2012.
- 6CMC Biotech Working Group. A-Mab: A Case Study in Bioprocess Development. 2009.
- 7CMC-Vaccines Working Group. A-VAX Case Study: Applying Quality By Design to Vaccines. 2012.
- 8Quality by Design for ANDAs.An Example for Immediate-Release Dosage Forms. FDA, 2012.
- 9Quality by Design for ANDAs.An Example for Modified Release Dosage Forms. FDA, 2011.
- 10QbD as an Improvement Methodology for Pharmaceutical Legacy Products. A Case Study. ISPE Spain A¬ffiliate. 2014.
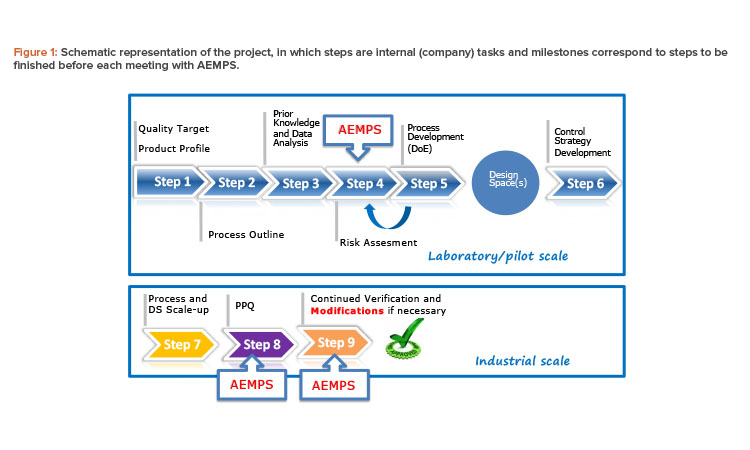
Scientific Advice Meetings
The formal meetings with AEMPS were categorized as scientific advice meetings. This categorization of the meeting was proposed by AEMPS given that throughout the project, extensive statistical data treatments were foreseen, as well as the use of novel analytical techniques. This way, the data would be evaluated while being generated, making it easier to evaluate the final documentation that would support the formal variations, once applied for. The first formal meeting was organized to review the proposed experimental strategy, mostly the type of experimental designs, variables, and corresponding levels and ranges to be studied, to support the creation of the corresponding design space. For that purpose, all historical data were previously statistically assessed for the whole period since the first commercial batch to provide information about the current manufacturing process’s state of control and identify the improvement needs.
Generally, the manufacturing process was under control. However, it was found necessary to improve the bioburden analysis sampling strategy, because isolated out of specification (OOS) results were obtained for this in-process control. The root cause in all occasions was found to be sample manipulation in a grade C environment. For this purpose, and in collaboration with the filter provider, a new filtration system was designed: a preassembled and gamma-irradiated system that contains sampling bags with aseptic disconnectors to avoid any sample manipulation prior to its analysis. Furthermore, the overall sterility assurance is increased by design.
Quality Assessment
An extensive and comprehensive risk assessment was performed to define the following:
- Quality target product profile (QTPP), critical material attributes (CMA), critical quality attributes (CQA) of the intermediate products of each unit operation and of the finished product, and the critical process parameters (CPP) (For CQA and CPP examples, see Tables A and B, respectively.)
- Experimental strategy for all manufacturing unit operations
Critical Quality Attribute | Surrogate Parameter |
---|---|
Appearance | Visual appearance |
Degree of color | |
Identity | Match the product main molecule |
Match the salt | |
Appearance of reconstituted solution |
Opalescence |
Absorbance | |
Reconstitution time | Reconstitution time |
Finished product pH | pH |
Residual moisture content | Residual water |
Purity | Related compounds |
Main compound assay | |
Extraneous particulate contamination | Subvisible particles |
Visible particles | |
Sterility | Sterility |
Pyrogenicity | Bacterial endotoxins |
Hermeticity | Tightness |
For the freeze-drying process, as an example, it was found necessary to investigate the impact of the variability of the CPPs (such as the shelf temperature and chamber pressure, once the endpoint of the sublimation was guaranteed) on the product quality, for all CQAs that may potentially be affected, such as aspect, reconstitution time, purity (assay and related compounds), and residual moisture content (RMC). The current freeze-drying cycle, as described in the dossier and applied in routine, had only fixed set points, and no structured data were available for any other combination of the set points. Taking into account that energy input changes from one freeze-dryer to another, even with theoretically same set-point values, and that the future freeze dryers (new manufacturing area) will be loaded by an automatic loading and unloading system, thus without trays, the risk of failure (affectation of one or more CQAs) due to the modified energy input was considered high.
A detailed experimental strategy was presented to define the experimental region. AEMPS suggested that a list of all possible Design of Experiments (DoE) were presented, along with the one chosen, to better describe the benefits and drawbacks of each potential DoE matrix. The aim was to find the most appropriate DoE that could maximize the significant information while reducing the number of experimental runs. This was completed also taking into account the duration of freeze-drying processes (more than 2 days, in this case). Further, it was proposed and accepted to study primary drying (sublimation phase) and secondary drying desorption phase) separately. This was done by first assessing the impact of the variability of the CPPs related with secondary drying (temperature and duration) while keeping the primary drying conditions fixed (those corresponding to the already approved and routinely applied for this product).
A total of three runs was necessary (at three different temperatures for secondary drying, with a 10°C difference among runs). The pilot-scale freeze-dryer was equipped with a sample thief, so in each run it was possible to extract samples at different timepoints without disturbing the process and while maintaining the process conditions as unchanged (apart the secondary drying duration) of all samples from the same run. The assessed CQAs were RMC, purity (assay and related compounds), and appearance (to evaluate the possibility of collapse during secondary drying, being the product amorphous). The duration range was set between 3 h and 16 h. The maximum evaluated duration was way above the current routine duration, but it was decided to assess the impact of additional time that may be added due to operational reasons (currently, night shifts have limited number of permitted operations).
Unit Operation | CPP |
---|---|
Compounding | Stirring speed |
Stirring time | |
Cooling jacket temperature | |
Final volume adjustment | |
Filtration | Filtration pressure |
Filtration temperature | |
Filter-solution contact time | |
Filtration time | |
Solution collection | Cooling jacket temperature |
Filling and pre-stoppering | Pump volume setting |
Nitrogen blowing pressure | |
Filling speed | |
Freeze-drying | Loading temperature |
Soak time | |
Freezing temperature | |
Freezing time | |
Primary drying time | |
Primary drying pressure | |
Primary drying shelf temperature | |
Secondary drying time | |
Secondary drying pressure | |
Secondary drying shelf temperature | |
Nitrogen backflush pressure | |
Crimping | Crimping station height |
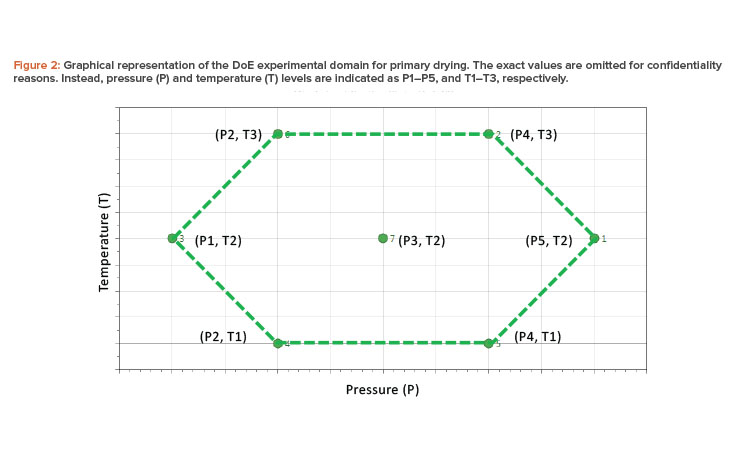
In all the cases, the CQAs complied with the specifications, and no significant variability was observed among runs and extractions. All the results of RMC were comprised between 0.4% and 0.8% (specification: NMT 5%). Likewise, the purity (assay, and individual and total related impurities) was not affected by increasing temperature and process time within the experimental region. No shrinkage was observed, so collapse during secondary drying did not occur. It was also confirmed, in evaluating the reconstitution time, that it may increase due to collapse either in primary or secondary drying. These results permitted the conclusion that the experimental region also represented the design space region for this part of the freeze-drying process at pilot scale. For further studies, i.e., DoE for the primary drying, the center point conditions for the secondary drying temperature and the shortest duration were set as fixed.
The following experimental task was the assessment of the impact of primary drying CPPs variability on selected CQAs, such as appearance (collapse) and reconstitution time. At the same time, with primary drying being the longest step, it was decided to evaluate the impact on the duration, with the aim of choosing the shortest, and thus most cost and energy-effective process, while guaranteeing quality. Figure 2 provides the Doehlert DoE experimental domain representation.11 , 12 This kind of DoE permits the study of two factors, pressure and temperature; in this example, at different number of levels, five and three, respectively. It is also possible to extend the experimental domain in different directions, and new factors may be added, if necessary. The estimation of main effects and all first-order interactions, as well as quadratic effects, is possible without confounding effects. The central point conditions coincided with the currently established conditions that had been set during the initial development, based on the thermal characteristics of the solution, such as glass transition of the freeze concentrate and the collapse temperature, assessed by differential scanning calorimetry and freeze-drying microscopy, respectively. These conditions were used for the three replicates to assess model quality.
After the nine experimental runs, and analysis of the corresponding CQAs, it was concluded that the experimental region could also describe the design space for the primary drying part of the freeze-drying process at pilot scale. As no statistically significant differences were found as outcomes of all cycles, it was not possible to model the CQAs as function of the CPPs. However, significant differences, in the range 16 h to 30 h, were observed in terms of process duration, as a function of pressure and temperature.
Along with the freeze-drying studies, experimental strategy was also applied to elucidate the impact of variability on other unit operations, such as compounding. In this case, CPPs that were varied systematically were stirring speed and temperature, to evaluate their impact of solubilization kinetics, foam formation, and degradation. Also, the new sterilizing filtration system was subject to experimental studies, in collaboration with the filter provider.
Data Evaluation
Once all the experimental work regarding the manufacturing process was finished, a comprehensive data evaluation was performed. It permitted the reassessment of the initial risk designation for each unit operation, taking into account the new findings. The aim was to describe the mitigation actions, including the pilot-scale design space (for freeze-drying) and normal operating ranges (for other unit operations), and to propose a formal risk-based control strategy for the scale-up exercise, for all unit operations.
After defining the control strategy and corresponding sampling plan, a full industrial-scale batch was manufactured to verify the newly developed process and corresponding design space. For freeze-drying, the proposed strategy for design space scale-up consisted of applying a cycle at the upper edge of the design space (P4 + T3, Figure 2) for primary drying, and at the lowest temperature and shortest duration of secondary drying. The rationale is based on the fact that all other, less aggressive, primary drying conditions (combinations of pressure and temperature) will yield satisfactory results if the upper edge is proven acceptable. For secondary drying, the combination of temperature and duration was chosen as possibly worst case in terms of desorption effectiveness and uniformity throughout the freeze-dryer. In all the cases, the endpoint of each phase was guaranteed by appropriate process analytical tools, the same as those used at pilot scale. For all unit operations, process data were collected continuously and compared with the corresponding acceptable ranges.
The analytical results, related with all CQAs, complied with specifications and were comparable with those at pilot scale. This confirmed that the design space could also be successfully applied at the industrial scale. At this stage, the first phase of manufacturing process validation 13 was considered finished and data were shared with AEMPS during the second formal meeting. At the same time, a process performance qualification (PPQ) strategy proposal was submitted for preevaluation. It was based on the risk reassessment after process scale-up. The number of full-scale batches to be manufactured was defined as at least three, where the final number of batches necessary to define all process phases as qualified would be based on statistical evaluation of the data to assess intra and interbatch variability and process capability. 14
PPQ Exercise
An extensive sampling plan was proposed for secondary drying (RMC mapping), the only manufacturing phase where the residual risk of lack of uniformity among vials was still considered medium. Given the batch size, the number of samples (315 per N batches) was very high, considering the analysis by Karl Fisher titration. Therefore, an alternative analytical method, based on near-infrared spectroscopy, was developed and validated. It permits analyzing hundreds of samples in a very short time without any sample manipulation, allowing effective evaluation of manufacturing process quality without errors due to sample manipulation. Furthermore, as the analysis is not destructive, it can be used to follow the evolution of the same sample during stability studies, or to correlate RMC and other CQAs.
The PPQ exercise was executed in accordance with what had been agreed upon with AEMPS. For this purpose, three full industrial-scale batches were sufficient to qualify all manufacturing phases. The freeze-drying process that was applied for PPQ, and later in routine manufacturing, was within the design space, close to the conditions of the scale-up batch (pressure 10% lower; temperature 12.5% lower), to minimize the duration and permit for a safety margin. For the PPQ exercise, two freeze-dryers were used (one for each full-scale batch of bulk solution) to assess the impact of different freeze-dryers on the process and product quality. All CPPs were demonstrated to be under control; likewise, the CQAs complied with the specifications. The RMC mapping showed very good uniformity (0.4% to 0.8%, specification NMT 5%). The two freeze-dryers showed statistically significant differences in terms of RMC, but after the evaluation, it was concluded that there was no practical difference, i.e., no impact on product quality, between the processes performed in the two freeze-dryers. This permitted to further downgrade the residual risk identified for secondary drying to low. Thus, the control strategy proposed for routine manufacturing for this CQA was reduced to only three vials, randomly sampled from any of the positions in the freeze-dryer.
Variations Evaluation
After executing the three PPQ batches, the data were summarized and shared during a third and final formal meeting with AEMPS. At the same time, the Continued Verification Strategy and a proposal of a PACMP were presented to AEMPS. The PACMP was prepared to anticipate the following:
- Change of the vial type from molded to tubing, with consequent further increase of the batch size (in the manufacturing area)
- Change of the manufacturing area with new and larger freeze-dryers, thus an additional increase of batch size
For each proposed future change (second group of variations), the exact aim was defined and agreed upon, as well as the failure mode and possible effects on the product quality. For every identified failure mode, necessary actions and experimental strategy were defined and the deliverables established, with the aim of downgrading the corresponding variation type. After adjusting the final text of the PACMP, it was submitted along with all other variations for evaluation. Given that the submitted documentation was previously evaluated during the formal meetings, the number of allegations was very reduced and these were focused on the dossier sections where the data were included, rather than the content or quality of the provided information. All variations, including the introduction of the design space, the routine use of PAT tools (such as endpoint determination for each batch by Pirani vacuum gauge), novel analytical techniques, and the PACMP were approved for routine implementation in only 6 months, significantly faster than in traditional applications where no previous communication with AEMPS is established.
This was an example of a win-win strategy. The same group of variations was presented in other European agencies (national procedures) and worldwide. The evaluation time was significantly longer in other European countries, with several groups of allegations in each of them, mostly focused on the definition of criticality and PACMP. In all cases, the variations were approved. On the other hand, in most non-EU countries, the evaluation was significantly different due to lack of expertise about QbD terminology and principles, and/or absence of regulatory harmonization. Although the same documentation, adapted to regional requirements, was provided in all cases, it was not possible to obtain the approval of the PACMP in most non-EU countries.
Conclusion
It is clear that better harmonization among regions and countries is still required so innovation can be implemented in the life cycle management of pharmaceutical products. Therefore, any initiative in this field, such as the announced ICH Q12 guidance, 15 will be very helpful.
- 11Doehlert, D. H. “Uniform Shell Designs.” Applied Statistics 19, no. 3 (1970): 231–9.
- 12Dejaegher, B. and Heyden, Y. V. “Experimental Designs and Their Recent Advances in Set-up, Data Interpretation, and Analytical Applications. Journal of Pharmaceutical and Biomedical Analysis 56, no. 2 (September 2011): 141–58. doi: 10.1016/j.jpba.2011.04.023
- 13United States Food and Drug Administration. Process Validation: General Principles and Practices—Guidance for Industry, 2011.
- 14Willes, F. “Risk-Based Methodology for Validation of Pharmaceutical Batch Processes.” PDA Journal of Pharmaceutical Science and Technology 67, no. 4 (July--August 2013): 387-98. doi:10.5731/pdajpst.2013.00923
- 15ICH Harmonised Guideline (Draft Version). Q12: Technical and Regulatory Considerations for Pharmaceutical Product Lifecycle Management. ICH, 2017.