Phase III Clinical Trials - Ever Wonder Why Some Products Unexpectedly Fail?
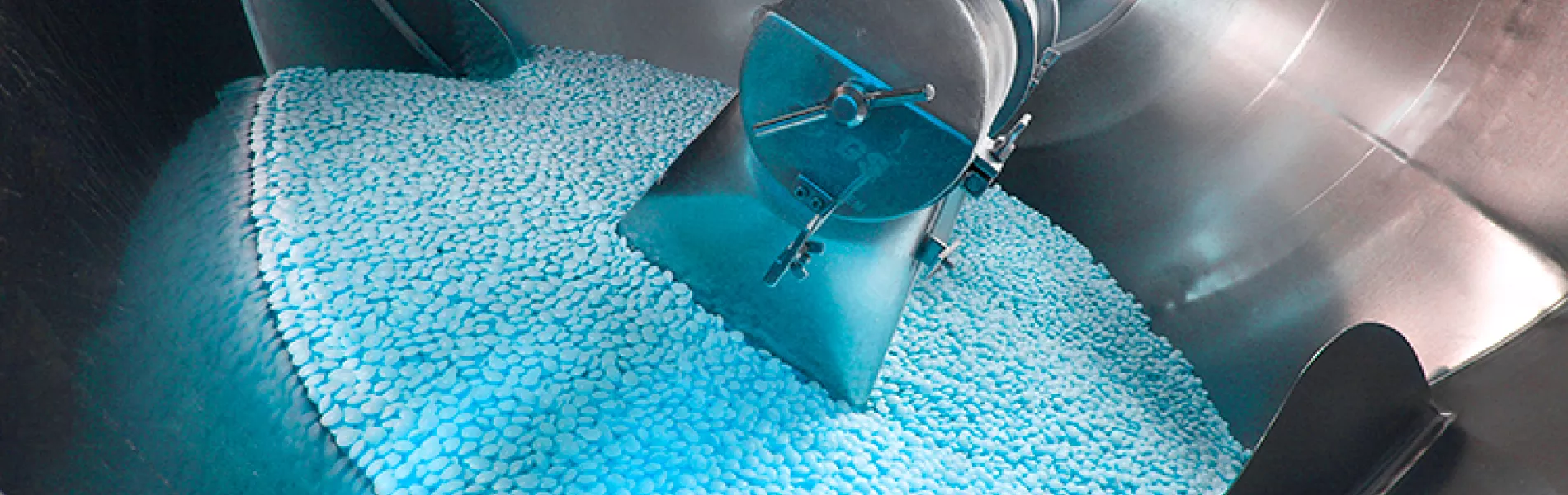
A fundamental flaw in the way the industry develops manufacturing processes could be the reason.
After a huge investment of time and money, a promising product with a sound therapeutic rationale shows initial safety and likely efficacy in Phase II clinical trials, then BOOM it fails in Phase III clinical trials. One reason is obviously that more clinical data shows that the product does not work. However, a second, a more insidious explanation may be possible from a regulatory agency induced fundamental flaw in the way biopharmaceuticals are developed. What if the product used in Phase III clinical trials was not the same as the product used earlier despite meeting the same product release specifications?
While defining a product in terms of CQAs (Critical Quality Attributes) is very important, complex biopharmaceuticals that include monoclonal antibodies, cellular therapies, etc. have a wide variety of Unknown CQAs (U-CQAs) that are neither understood nor measurable. These U-CQAs may play as much a part of determining the safety and efficacy of a product as the CQAs. The way the industry currently develops biopharmaceuticals does not place sufficient emphasis on, nor provide adequate design tools for controlling U-CQAs. If U-CQAs are not adequately controlled during the product/process’s development and manufacturing lifecycle, some products will undoubtedly fail phase III clinical trials because they are not testing the same product.
So, what is the flaw? You don’t hear the old industry cliché “the process defines the product” much anymore. The more complex the biopharmaceutical the truer the quip. The cliché came from an early, but apparently mostly lost, appreciation of how little was really known about biopharmaceuticals. So, how do you control U-CQAs?
Controlling the process is the only reasonable method of controlling U-CQA by controlling the process’s behavior during the formation of all the product’s quality attributes as it is manufactured. The current approach to controlling product quality is primarily focused on controlling the measurable CQAs with little attention paid to controlling all of the possible product quality attributes over the entire product development lifecycle.
The root cause of the problem can be found in ICH-Q8’s weakly defined Design Space (DS) described in terms of CQAs and very poorly defined CPPs (Critical Process Parameters).1 ICH Q8’s CPP definition covers both input and output parameters. Clearly describing a process’s inputs and outputs along with their interactive relationships is fundamental to developing effective control systems. While the CPP/CQA defined DS is sufficient for regulatory purposes, it is woefully inadequate for developing biopharmaceuticals and building the necessary product quality Control Strategies (CS) for controlling both CQAs and U-CQAs.
A manufacturing process has two outputs. One is obviously the product it produces (CQAs and U-CQAs). The other outputs are a variety of process parameters, many of them easily measurable, that describe the process’s behavior and performance. Borrowing a term from Design of Experiments (DOE), these output parameters can be called Critical Process Responses (CPRs). In the rare case that a CQA can be measured in real time, it may be used as a CPR. Since U-CQAs cannot be measured, the only way to control how they are formed is to measure and control the process’s CPRs. For example, a process’s yield, a CPR, is well known to frequently have a significant impact on the product’s CQAs and thus very likely to also impact U-CQAs. While much data may show yield has little impact on CQAs, the perhaps more important question of whether yield impacts U-CQAs will forever remain unanswered. While certainly no guarantee, tight control of CPRs provides the only method of potentially comprehensively controlling product quality.
To make matters worse, CPPs are also a jumble of different kinds of input parameters commingled with some output parameters leading to confusion. Many companies struggle with untangling and clearly defining the mixture as the process sequence is designed. Some examples of developers not knowing if a parameter is an input or an output from a specific process have been seen.
From a careful study of all the different types of input process parameter, three very distinct categories can be identified. The first is material input parameters (MPs). These inputs are occasionally and inconsistently labeled as Critical Material Attributes (CMA). MPs describe the attributes of the material inputs to the process. An example of an MP might be the purity of media components and salts used to make buffers.
A second category is equipment input parameters (EPs) usually defined during the selection of equipment for executing the process. The EPs are frequently stated in the equipment’s User Requirement Specifications (URS) when the equipment is purchased. EPs and their impact should be clearly understood and carefully controlled during scale-up as the capacity of the process is increased. Examples of EPs might include tank volumes, materials of construction, agitator types, and heat transfer surface areas and characteristics.
The third, and possibly the most important, are the operating input parameters (OPs) used to control the process’s behavior to produce the product. Many OPs are set during process development (e.g., buffer concentrations, agitator speed, etc.) while others are actively controlled during manufacturing such as flow rates, concentration gradient rates, etc. Of great importance is that all three types of input parameters require very different control strategies.2
The two types of process outputs and three types of process inputs can be used to build a well structure Design Space (ws-DS) as a foundation for controlling all of the product’s quality attributes, both known and unknown. For those attributes and parameters, the ws-DS can be briefly described as2 :
- Process Responses (PR) – CPRs are important output measures of a process’s behavior and performance.
- Quality Attributes (QA) – CQAs are important measurable attributes of product quality.
- Material Parameters (MP) – CMPs are important measurable attributes of the input material quality.
- Equipment Parameters (EP) – CEPs are important input parameters that define the equipment and instruments. Most EPs are stable, but a few CEPs can change over time and should have active control systems, usually through effective monitoring (CPRs) and maintenance.
- Operating Parameters (OP) – COPs are important input operational and control parameters used to control the performance of the process. Many COPs are set during development while others may be used for active control using feedback loops.
The ws-DS can be defined for specific unit operations (UOs), groups of UOs, or an entire manufacturing process. More detailed definitions, including their control systems and strategies can be found elsewhere.2
However, the complete ws-DS also describes the relationships between the five elements of the ws-DS and their interactions to form the necessary CSs for controlling product quality. Many CPRs are linked to and controlled by a COP. COPs that are actively use in control loops to control CPRs are frequently called Critical Control Parameters (CCPs). The real-time performance of both CPRs and CCPs can provide invaluable tools for controlling all the product quality attributes.
A consistently defined and used ws-DS would have significant benefits to the developing company and regulatory agencies by building a uniform foundation for developing and reviewing process information to make sure that all the product’s quality attributes are controlled over the entire product development and manufacturing lifecycle. If ICH M4 – CTD (Common Technical Document) sections were defined using a ws-DS/CS structure, then manufacturing processes could be more effectively designed and communicated.
So, the next time a valuable promising product mysteriously crashes and burns in Phase III clinical trials, ask the questions: Was it the same product? And, was the product’s complete Design Space properly developed and controlled during the product’s entire manufacturing lifecycle? Or better yet, ask the questions before the product start its phase III clinical trials.
- 1FDA (CDER/CBER) – Guidance for industry: Q8(R2) pharmaceutical development. Nov 2009. ICH, Rev 2.
- 2 a b c Witcher MF, Integrating Development Tools into the Process Validation lifecycle to achieve six sigma pharmaceutical quality. BioProcess J, 17 (2018). https://doi.org/10.12665/J17OA.Witcher.0416