A Sustainable Approach to Steam Quality Management

The world is beginning to grasp the huge challenge of achieving net-zero carbon emissions, or carbon neutrality, by 2050. Many countries have committed to achieving this ambitious goal. As a major global industry, the pharmaceutical sector has a significant role to play. For thermal energy–intensive industries, such as pharmaceutical manufacturing, the long-term future options to maintain current manufacturing processes are few and look to signifi cantly increase energy costs. Optimizing current systems and considering new strategies is necessary.
UNDERSTANDING THE CHALLENGE AHEAD
Net zero is defined as when the amount of carbon dioxide we add is no more than the amount taken away. Whether electrification, hydrogen, biofuel, or even synthetic fuels are considered, each will require significant investment in production, distribution, and infrastructure at a national, local, and individual site level to make the necessary changes.
The journey to achieve net zero must therefore include our focused and deliberate efforts to minimize infrastructure investment cost and timeline to delivery. This can be done by treating energy as a highly valuable resource and establishing best practices in energy efficiency, eliminating waste, and recovering and reusing waste heat from existing processes.
Categorization of Greenhouse Gas (GHG) Emissions
GHG emissions are categorized into three groups or “scopes” by the most widely used international accounting tool, the Greenhouse Gas Protocol1 . Scopes 1, 2, and 3 are a way of categorizing the different kinds of carbon emissions a company creates within its own operations, and across its wider value chain (see Figure 1).
It is possible to think of scopes in terms of three categories of emissions—scope 1, 2, and 3—and key themes emerge when considering the scopes.
Scopes 1 and 2
Scope 1 covers the GHG emissions that a company makes directly; for example, while running its boilers (including steam), manufacturing utilities, vehicles, etc. Scope 2 covers emissions a company produces indirectly or emissions produced on the company’s behalf. These include emissions from electricity, steam, and energy purchased for heating/heat transfer, cooling buildings, and manufacturing utilities.
Scope 1 and 2 emissions are usually within an organization’s control. It is highly likely that an organization will have access to the source data needed to convert direct purchases of gas, steam, and electricity into a value in tons of GHGs. This information may be captured and managed by procurement, finance, estates management, utilities, or facilities management departments, or in a sustainability function.
In some cases, solutions currently exist to deliver net zero for scope 1 and 2 emissions. For example, an organization can source renewable electricity, gas, and steam; electrify the source of its energy transfer/heat demand (or use another suitable renewable option); and transition to electric vehicles.
Scope 3
This scope covers all of the emissions that the organization is indirectly responsible for, both up and down its value chain. For example, an organization is indirectly responsible for the emissions produced from purchasing products from its suppliers and from its products when customers use them. Scope 3 emissions are a highly impactful concern.
Scope 3 emissions tend to have the most negative impact and are more challenging to address. For many businesses, scope 3 emissions will account for more than 70% of their carbon footprint. For example, for an organization that manufactures products, there will often be significant carbon emissions resulting from the extraction, manufacture, processing, and distribution of the raw materials and finished goods.
Organizations may also have less control on how scope 3 emissions are addressed. Businesses can offer to collaborate on solutions to reduce emissions with current suppliers or consider changes to their own supply chain. However, in most areas, businesses’ current suppliers will have considerable influence on how emissions are reduced through their own purchasing decisions and product design.
- 1World Resources Institute and the World Business Council for Sustainable Development. “Greenhouse Gas Protocol: Technical Guidance for Calculating Scope 3 Emissions (Version 1.0).” 2013. https://ghgprotocol.org/sites/default/files/standards/scope3_Calculation_Guidance_0.pdf
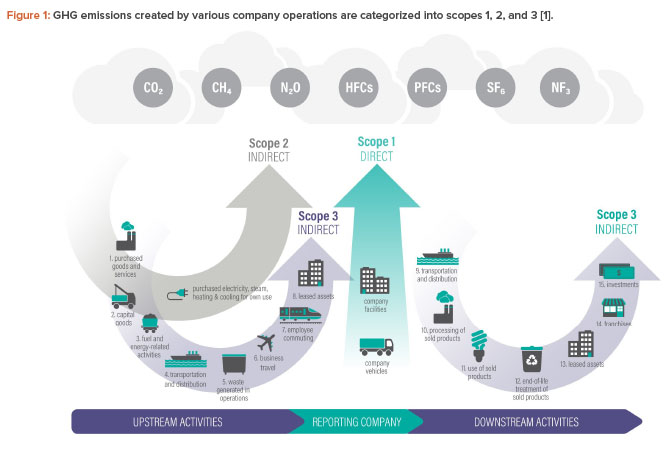
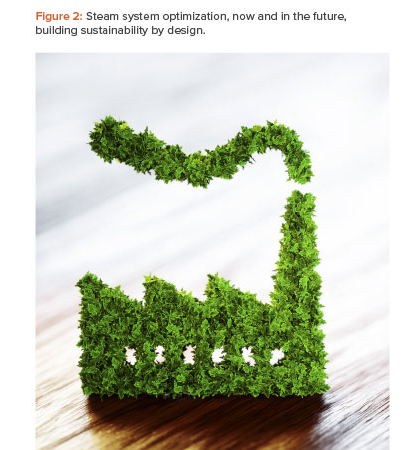
Committing to reach net zero will involve significant focus on scope 3 emissions. Definitions for what constitutes net zero ambition are evolving, but businesses looking to adopt best practices will commit to focusing on scope 3 emissions as part of their plans. Mapping a company’s emissions footprint by scale, and how much control a company will have over the source of such emissions, will be an effective way to start addressing them. It will also bring the emissions hotspots within reach to effect change.
WHY THE PHARMACEUTICAL INDUSTRY USES STEAM
It is prudent to specifically address the pharmaceutical industry’s need to raise, distribute, and bring steam of the correct quality and quantity to each plant and process point of use (POU). It is the intent of the authors that the following discussion may be considered as an example of how such steps could be taken when considering scope 1 and 2 emissions within the pharmaceutical industry. Equally, it may be considered that such principles and examples may be applied to other industries that currently use steam as part of their manufacturing requirements. Together, we have significant opportunity to optimize existing steam systems and innovate new ones, building sustainability by design now and in the future (see Figure 2).
In discussing the use of steam in the pharmaceutical industry, we should first ask, “Why is steam used in pharmaceutical manufacturing across plant and process (critical) utilities?” The answer is simple, but offers a very compelling and insightful outcome. Steam is powerful, and it has capabilities that other fluids will find hard to match. The high-energy density of steam enables efficient and effective thermal energy transfer with precise temperature control (through steam’s pressure/temperature relationship).
There are two important factors to consider as we continue to explore steam’s role in pharmaceutical manufacturing: First, it is necessary to ensure existing steam systems operate as efficiently as possible for the energy employed. Second, it is necessary to ensure steam of the correct quality and quantity arrives at each plant and process POU, thus enabling a safe, compliant, and energy-efficient system, or sustainability by design.
Steam is widely recognized as a powerful and highly efficient thermal energy transfer fluid. Steam itself is a natural fluid and environmentally benign; when all of the available heat from steam is used, only water remains in the form of condensate, which can be reused through the steam and condensate loop. However, the current general method used to raise steam is burning fossil fuels, which means steam generation is a significant source of carbon intensity.
Steam Quality Management Enables Energy-Efficient Systems
From the boiler through distribution to plant and process POU, it is critical to achieve a stable, mechanically correct, and appropriately maintained steam system by adhering to steam system engineering best practices. This will ensure steam of the correct quality and quantity is raised, distributed, and provided to each POU (plant and process/critical utilities).
Then, one can consider further optimization of the system. In short, each POU is 100% reliant on steam of the correct quality and quantity to ensure efficient and appropriate energy transfer to the manufacturing process.
One such example is heating, ventilation, and air conditioning (HVAC) systems. HVAC systems have always been an integral part of pharmaceutical manufacturing across comfort and current Good Manufacturing Processing (cGMP) areas. Many large pharmaceutical multinational companies estimate that HVAC demands account for 60%–70% of total operational energy consumption. In fact, operating costs of cGMP HVAC in cleanrooms are often 20–100 times larger than those employed in typical comfort areas, such as offices.
With such large numbers in mind, it is understandable that reducing overall consumption and improving efficiency is an area of importance for utilities and facilities management teams. Indeed, there has been considerable focus on demand reduction, and the complete steam system represents a compelling area where efficiencies may be identified and implemented.
Therefore, it is critical for organizations to embed stepwise and pragmatic sustainability initiatives into day-to-day improvement operations, as well as the overall business model. This will enable positive and impactful change, which can be felt across the entire pharmaceutical community.
BACKGROUND ON STEAM QUALITY MANAGEMENT
At this point, it may be helpful to frame steam quality as the measure of steam’s thermal energy transfer attributes and the relevance of the globally recognized EN 285 standard and methodology2 . First, we discuss the different steam types and their manufacturing purpose and suitability.
Steam Types
Pharmaceutical steam may be classified into two types based on their respective sources:
- Plant steam, raised from a utility boiler: A universally used steam grade for efficient thermal energy transfer
- Process steam, generated from a nonutility dedicated source:
- Chemical-free steam: A nonproduct contact grade used in nonsterile manufacturing
- Pure steam (clean steam): A direct product contact grade used in sterile manufacturing
Plant steam
Plant steam is normally produced using conventional steam boilers, typically of steel construction, and potable water, with chemical additives to raise the pH to 9.5–10.5 to protect carbon steel equipment from scale and corrosion. These scale and corrosion inhibitors may include substances, such as amines (that cannot be removed through filtration) that may not be acceptable in steam used in pharmaceutical processes (e.g., form a contaminant, which may cause an undesired reaction in the drug product). Hence, plant steam is used in applications that do not involve direct contact between the steam and the product or product contact equipment.
Plant steam is raised at relatively high pressure with the potential of generating superheat during expansion. Superheated steam is produced by heating the steam beyond saturation temperature or by generating the steam at higher pressure in a boiler and then reducing the pressure through a regulating valve. Superheat may be dissipated downstream of the regulating valve due to heat loss in distribution pipework.
The presence of superheat makes steam more difficult to condense, as a portion of the heat exchange surface will be used to remove the sensible heat before a phase change can occur. The presence of superheat may be beneficial when considering steam distribution, but problematic when considering efficient thermal energy transfer points of use, such as heat exchangers and sterilization processes.
Plant steam is used as a heat source for noncritical and cGMP heat exchangers for heating (frost protection) coils in HVAC applications, as well as in critical applications such as water for injection (WFI) production via sanitary (double tube sheet) heat exchangers. Plant steam can also be used for biological destruction of nonproduct contact equipment or of solid or liquid wastes in equipment, sometimes known as kill tanks.
Chemical-free steam
Chemical-free steam is a noncontact steam grade. It is produced by a steam generator from pretreated water with nonvolatile additives that meet the FDA Generally Recognized as Safe (GRAS) listed additives or an equivalent international standard, where applicable. It is primarily reserved for humidification and nonsterile product sanitization or bioburden control.
Chemical-free steam is also used for noncritical steps in the manufacture of active pharmaceutical ingredients (APIs) without product contact and humidification for HVAC pharmaceutical systems (usually provided prior to the system’s high efficiency particulate air [HEPA] filter). The bioburden control of early-stage manufacturing equipment both fall into this category. Chemical-free steam provides an acceptable level of purity because any added impurities will be removed in subsequent procedures.
Pure steam
Pure steam is a direct contact steam grade produced by a steam generator. When condensed, the steam condensate meets the requirements of relevant compendial standards (e.g., US Pharmacopeia [USP], European Pharmacopoeia [Ph. Eur.]) for WFI, except for microbial content (steam purity in chemical and biological composition).
Pure steam is predominantly used for sterilization. Steam used for sterilization in autoclaves for international manufacturing also should meet the requirements of the EN 285 standard and methodology. This will ensure steam’s thermal energy transfer attributes (i.e., dryness, superheat, and noncondensable gases), all of which negatively impact the efficient thermal energy transfer properties of steam, are rigorously maintained.
When considering pure steam, guidance documents from regulatory agencies include various definitions. The commonly used terms are “pure steam” and “clean steam.” In this article, we use the term “pure steam,” as referenced in USP 1231 “Water for Pharmaceutical Purposes”3 .
Pure steam is generated from treated water that meets applicable drinking water regulations and is free of volatile additives, such as amines and hydrazines (which can react with pharmaceutical products). It is used for sterilization processes and is considered especially important to mitigate contamination risk from injectable drug products.
Pure steam is characterized as having no additives or buffer and limited generated superheat except when the generated pressure is significantly higher than the use pressure of the steam. The condensate of pure steam should meet the requirements of relevant compendial standards (e.g., USP and Ph. Eur.) for WFI (except for microbial content), and should not contain additives or buffer, with a relatively low pH compared to that of plant steam.
The user has the ultimate responsibility for system design and performance and for ensuring that the proper type of steam is used for a given POU/process. The USP provides guidance as to the generation, quality attributes, and uses of pure steam3 . The pure steam section of the USP monograph provides direction for the feed water source, added substances, and testing of condensable attributes. Pure steam dryness and noncondensable gases, however, should be determined by the user based on the intended POU, guided by the globally recognized EN 285 standard and methodology.
The parameters used for confirming the quality of pure steam used for sterilization can also be used to measure the quality of other steam types. The test results can be interpreted based on the use of the steam at that point. The parameters to be considered are dryness, superheat, and noncondensable gases.
Further discussion on these parameters and their potential impact is included in the Pharmaceutical Engineering® article “Introduction to Steam Quality and Testing”4 . The EN 285 standard provides guidance on the test methods for these attributes, with specialist companies offering internationally recognized vocational qualification in these testing parameters (e.g., City & Guilds).
Steam Management Industry Practices
Steam for sterilization
When steam or the resulting condensed water comes into direct or indirect contact with the drug product, the purity (i.e., the correct grade of steam) should be equivalent to the water purity acceptable for the manufacture of the drug product (i.e., purified water and WFI).
It should be noted that a continuous supply of dry saturated steam at the POU is considered necessary for efficient steam sterilization. As discussed previously, water carried by steam in suspension may reduce heat transfer. Superheated steam is considerably less effective than saturated steam when used for sterilization, or any other POU where efficient thermal energy transfer is required. Noncondensable gases, if contained in the steam, act as an insulating barrier to efficient thermal energy transfer and may prevent the attainment of sterilization conditions in parts of the sterilization load.
Steam POU | Method of Steam Generation or Steam Grade |
---|---|
Sterile dosage form applications where steam may be in direct drug product contact with product/product contact surfaces | Pure steam generator or multiple-effect still |
Critical step in the manufacture of APIs used for sterile drug product that may be in direct drug product contact with the API/API contact surfaces | Pure steam generator or multiple-effect still |
Humidification of cleanrooms systems, where the drug substance/product/primary container is not directly exposed to room atmosphere or where steam is injected pre-HEPA filter | Chemical-free steam generator (fed with potable or softened potable water) |
Humidification of cleanrooms systems, where the drug substance/product/primary container is exposed to room atmosphere and steam is injected post HEPA filter | Pure steam generator or multiple-effect still |
Heat sources for noncritical (nonproduct contact) and cGMP heat exchangers | Plant steam |
Deactivation of solid or liquid biological process waste (i.e., kill tanks) | Plant steam in a dedicated deactivation vessel: The use of a pure steam generator may be required if kill is performed within a process vessel |
Bioburden control of direct product contact production equipment, process vessels, and containers | Chemical-free steam generator (fed with potable or softened potable water) |
Sterilization of direct product contact production equipment, process vessels, containers, and packaged product | Pure steam generator or multiple-effect still |
Steam for humidification
When steam is used for indirect humidification, such as injection into HVAC air streams prior to final air filtration, the steam grade does not need to be purer than the entrainment air and suitable plant steam. It should be noted that chemical-free steam, produced from a generator fed with pretreated water and without buffers or corrosion inhibitors, is suitable for some HVAC humidification applications (i.e., nonsterile manufacturing).
When humidifying process areas, however, the potential level of impurities including amines and hydrazines should be evaluated to ascertain the impact on the final drug product. This is particularly important in areas where open processing takes place, such as aseptic filling suites and formulation areas. If the diluted water vapor is found to contribute to the contamination of the drug, a purer grade of steam should be selected.
STEAM’S THERMAL ENERGY TRANSFER ATTRIBUTES
From boiler to distribution and to each plant and process POU, a complete steam system audit will address the many areas that pharmaceutical facilities management teams may consider when looking to improve steam system efficiency. Some of these steps can be very simple.
For example, steam quality testing (which allows for understanding of steam’s thermal energy transfer attributes at critical test points) and steam trap surveys (which establish a complete inventory of all plant and process steam traps) are both steps that may improve steam system efficiency. Such information enables stepwise and pragmatic recommendations to be made based on steam system engineering best practice. This ensures a safe, compliant, and energy-efficient system that meets local legislation.
It should be noted that isolating dead legs (sections or components of a steam pipework system that have minimal or zero flow which can lead to stagnation, contamination, and corrosion of the fluid and the pipe) or pipework to processes that are not in use for a long period of time is considered good practice. Keeping distribution lines hot when not needed (e.g., steam lines to frost coils in summer) wastes energy both in terms of steam production and often again in the HVAC system when removing such heat from the production environment. If isolation valves are located at height or in a technical space, making it difficult for access, thought should be given to the use of actuated valves that can be operated remotely or as part of the building management system.
Steam pipe insulation condition has a significant role in reducing energy loss and reducing condensate levels in distribution piping. Often after a maintenance period, insulation and insulation jackets are removed for access and then are not replaced. This results in energy loss and excess condensate forming.
Note that poor insulation, dead legs, and suboptimal steam trap performance can result in the formation of additional condensate, which can affect the dryness measurement component at critical points. Therefore, steam quality testing at critical points can serve as a good qualitative measure of system performance and diagnostic indicator for thermal efficiency.
THE NET ZERO TRANSITION AND CHANGES TO BOILER ROOMS
Boiler rooms are responsible for large amounts of pharmaceutical emissions, and adjustments to boiler room operations will be necessary to reach net-zero goals.
Reduced Fuel Consumption
Fortunately, the boiler room presents several opportunities to reduce fuel consumption, such as by controlling a boiler’s total dissolved solids and using an associated heat recovery system. Another way to reduce boiler room fuel consumption is to capture thermal energy in the boiler flue gases using an economizer, which is typically used to preheat feed water going into the boiler.
Flash recovery from the condensate blowdown vessel can also be used to preheat water and reduce energy consumption. Fuel savings from each of these examples, which lower emissions while improving efficiency, can typically be between 3%–5%.
Electric Boilers and Energy Storage
Boiler manufacturers are already diversifying and offering both electric and hydrogen-fueled boilers. And as energy supplies become less flexible, energy storage will be key in providing uninterrupted process heat.
Electrically generated steam is 15%–20% more efficient than steam from conventional natural gas boilers and with zero flue gas emissions. Combining electrical heating within a boiler or steam generator and subsequent accumulator allows thermal energy, in the form of steam, to be stored when electricity is available or when it is available at low cost when there is oversupply in the grid. This allows for the system to store and discharge steam, as required by the users. It is also possible to charge and discharge at the same time, allowing the use of a smaller generation source with a system that is capable of meeting high peak demands that exceed the generator capacity, thus providing greater flexibility.
Another advantage is that such energy accumulation can be placed closer to the POU, reducing pipeline losses and maintaining steam quality. Furthermore, such technology lends itself perfectly to plant steam indirect heating/thermal energy transfer applications, using a standard accumulator vessel, as well as chemical-free steam used in nonsterile pharmaceutical manufacturing processes, using a suitable stainless steel accumulator vessel.
Such technology is important to demonstrate strong commitment to sustainable energy. It supports the pharmaceutical industry as it strives to reduce steam system carbon intensity, and it ultimately provides a zero-carbon energy source as the grid receives more sustainable energy from renewable sources such as solar, wind, hydrogen, and even nuclear.
Other Emission Reductions
Other possible options for emissions reductions in boiler rooms include generating steam from the combustion of organic waste materials, such as olive pulp, rice husks, and palm kernel shells created from various manufacturing processes. Biomass can be used to generate electrical energy as well as heat when used in a combined heat and power system.
The reduction of organic waste and the use of biomass helps improve environmental sustainability and lower energy bills. In addition, wood chip boilers are already used in the US, eliminating fossil fuels and enabling renewable resource usage. Flues are also controlled for stack emissions.
SUSTAINABILITY THROUGH ENERGY MONITORING SYSTEMS
Sustainability improvements are available with the use of energy monitoring systems. Such technology is invaluable to establish service demand and consumption patterns, enabling key stakeholders to know where, when, and how action should be taken.
Those responsible for energy use across the complete plant and process steam system often use burner efficiency to track overall efficiency, but this method does not consider all aspects of the boiler operation. Factors such as fouled heat transfer surfaces, carryover, radiation losses, and excessive boiler blowdown rates all impact overall boiler room efficiency. This, in turn, may lead to an overestimation of actual boiler room efficiency. It is critical to remember that plant steam quality has a direct impact upon process steam quality, due to the thermal energy transfer attributes of steam at critical heat exchange points, such as a sanitary heat exchanger prior to a pure steam generator or a WFI system.
Using dedicated methods and technology, a complete overview of the plant and process steam system is possible, allowing measurement of overall boiler room efficiency, distribution efficiency, and POU quality. This will help target improvements and associated savings. The utilities and facilities management team may consider using measured inputs from fuel, feed water, steam output, condensate return, and blowdown to build a true picture, helping yield energy reductions and increasing overall efficiency.
Some key considerations of measured inputs:
- Remote monitoring and control of steam systems will play a key role in sustainability.
- Over and above inefficient reactive responses to everyday problems, digitalization brings early alerts, predictability, and rapid problem-solving with new levels of control.
- Greater efficiency is built-in; problems are detected and acted upon immediately, before more severe consequences present.
- Early problem detection means smarter maintenance and repairs.
- Optimized steam systems yield a significant reduction of carbon intensity as energy emissions are reduced.
- Rigorous steam quality management across the complete system will enable improved process productivity through efficient thermal energy transfer.
A COMPLETE STEAM SYSTEM AUDIT APPROACH
Pharmaceutical utilities and facilities management teams may continue to make considerable progress with some of the steps mentioned previously. However, there will certainly be times when these teams will need to engage with specialists outside of their organization. Knowledge partners are available to provide expert advice through the deployment of steam system engineering best practice to ensure complete steam quality management through a complete steam system audit approach.
Steam system audits are vital tools to understand the health and wellbeing of the complete plant and process steam system. The audit assessment helps identify recommended improvements based on steam system engineering best practice and steam quality management. These changes ensure a safe, compliant, and energy-efficient system, while meeting stringent local legislation.
Steam System Audit Steps
The steps for the steam system audit follow. In addition, it should be noted while respecting the challenges of COVID-19 and social distancing, it is possible to conduct remote steam system audit capabilities with the use of digital technology (e.g., sensor data, video, and images) to identify areas for improvement.
Initial assessment
- Deploy steam quality testing capabilities (the authors suggest adopting EN 285 tests and methodology) to understand steam’s thermal energy transfer attributes at critical test points.
- Deploy steam trap survey capabilities to establish a complete inventory of plant and process steam traps, considering status, condition, maintenance record, and supporting validation/compliance documentation.
Comprehensive assessment
- Conduct a complete steam system overview, from boiler through distribution to each plant and process POU to identify energy saving possibilities and optimization solutions.
- The initial assessment and comprehensive assessment will focus on achieving a stable, mechanically correct, and appropriately maintained plant and process steam system, otherwise known as sustainability by design.
Thermal energy assessment
- Conduct detailed steam and condensate loop thermal energy mapping to discover plant and process optimization possibilities and to understand and address thermal energy demand reduction possibilities across the complete steam and condensate system.
- A thermal energy assessment approach is taken on a case-by-case basis, as the scope of such an assessment is often very broad with multiple variables and site-specific details.
Steam System Engineering Best Practice
Training onsite staff and optimizing their skillsets, focusing on steam system efficiency, can also contribute to a more sustainable operation. Seeking out training centers that provide accredited courses, such as the Combustion Engineering Association’s Guidance on Safe Operation of Steam Boilers (BG01) course, ensures safe working.
It also equips key personnel with the skills and knowledge, such as steam quality testing or EN 285 standard and methodology, required to maximize the efficiency and performance of the steam system. Again, as we adapt to a changing work environment, remote training and webinars are an increasingly effective way of ensuring that personal development can continue.
THE TRANSITION TO GREENER STEAM TECHNOLOGIES
More industries and organizations are recognizing that steam, as a highly efficient thermal energy transfer fluid, integrates with their sustainability initiatives. Adopting greener steam generation, distribution, and POU methods is a simple way to reduce GHG emissions. With new steam generation technologies, we are on a path toward carbon-free steam generation.
The current focus on climate change has brought into sharp focus the desire to invest in greener steam technology, as the world’s demand for heat continues to grow. Raising the efficiency of steam generation will make a significant sustainability impact in the long term, and electric steam generation systems, mentioned earlier in this article, would be one such example. Furthermore, the immediate sustainability impact will be realized through the attainment of stable, mechanically correct, and appropriately maintained existing steam systems, through the rigorous deployment of steam system engineering best practice and steam quality management.
Sustainable Solutions Are Available
Industrial-scale solutions are already in operation to generate, distribute, and deliver steam of the correct quality and quantity to POU in cleaner, more sustainable ways. Renewable, electric steam is carbon-free, emission-free, and 100% renewable steam.
- Higher-capacity users in the pharmaceutical sector can decarbonize their steam generation today, thanks to electric systems. Such systems align well with the portfolio of solutions driving progressive companies toward a truly sustainable business.
- When coupled with 100% renewable power sources (such as hydroelectric, PV solar, and wind), electric steam generators are capable of steam production without emission or carbon generation.
CONCLUSION
- As pharmaceutical manufacturers continue to deploy global sustainability initiatives to reduce their carbon footprint, the focus on energy-saving solutions is greater than ever. With particular focus on steam systems critical to manufacturing processes, steam users in the pharmaceutical sector should be reassured that there are various ways to lower emissions.
This can be done while improving efficiency, maximizing the impact of existing steam infrastructure, and working in a safe, smart, and sustainable manner. In working to lower emissions, pharmaceutical manufacturers will address the two considerations for steam’s role in pharmaceutical manufacturing:
- To ensure existing steam systems operate as efficiently as possible for the energy employed
- To ensure steam of the correct quality and quantity arrives at each plant and process POU, thus enabling a safe, compliant, and energy-efficient system
Taking this into account, a complete steam system audit approach to steam quality management, as previously mentioned, will enable pharmaceutical utilities and facilities leaders to meet sustainability goals. It will allow them to improve efficiency through the deployment of steam system engineering best practice and steam quality management. This will ensure maximum system performance for the energy employed and that steam of the correct quality and quantity arrives at each plant and process POU.
Steam is natural and part of everyday life. This extraordinary fluid is a highly efficient, mission-critical tool for diverse and important industries, such as pharmaceuticals, and is increasingly relevant as part of our sustainable future. Existing steam systems can be significantly optimized to perform far more efficiently than they currently do, and a complete steam system audit approach to steam quality management will enable a stable, mechanically correct, and appropriately maintained steam system to be achieved—the very foundation of sustainability.
- 2European Standards. “EN 285 - European Standards ILNAS EN 285+A1:2021, EN 285 Sterilization - Steam Sterilizers - Large Sterilizers.” November 2021. www.en-standard.eu/ilnas-en-285-sterilization-steam-sterilizers-large-sterilizers/
- 3 a b US Pharmacopeial Convention. USP<1231>: Water for Pharmaceutical Purposes. “Section 3.1.4 – Providing Guidance as to the Generation, Quality Attributes, and Uses of Pure Steam.” Rockville, MD: US Pharmacopeial Convention, 2017.
- 4Cohen, N., N. Haycocks, J. Miller, D. Mullins, and K. Shuttleworth. “Introduction to Steam Quality and Testing.” Pharmaceutical Engineering 42, no. 4 (July/August 2022): 54–63. https://ispe.org/pharmaceutical-engineering/july-august-2022/introduction-steam-quality-and-testing
- 5International Society for Pharmaceutical Engineering. ISPE Baseline® Guide: Water and Steam Systems (Third Edition). “Section 7.5.3, Industry Best Practices for the Production of Steam.” North Bethesda, MD: International Society for Pharmaceutical Engineering, 2019.