Reevaluating Transfer of RTU Containers Into Grade A

At first glance, ready-to-use (RTU) primary packaging material (tub systems) give production lines more flexibility and reduce container preparation complexity for aseptic fill/finish operations. However, the aseptic introduction of RTU tub systems requires a thoroughly designed transfer process to avoid contamination of the sterile RTU items and the aseptic core.
With more industry experience in technical transfer solutions and the release of the European Commission’s updated good manufacturing practice (GMP) guidelines, specifically Annex 1, the section on the manufacture of sterile medicinal products 1, it’s time to reevaluate transfer setups using a holistic quality risk management approach.
Introduction
“The transfer of equipment and materials into and out of the cleanrooms and critical zones is one of the greatest potential sources of contamination,” per the EU guidelines 1. RTU tub systems can serve as an alternative. They are commonly presented in nests or trays to avoid glass-to-glass contact. Depending on the type of pack style (i.e., syringe, vial, and cartridge), the containers are pre-assembled and pre-treated (i.e., washed and siliconized). RTU packaging material for aseptic processing comes pre-sterilized in their overpack, either by irradiation (gamma or beta irradiation, limited to plastic packaging material) or ethylene oxide gas treatment.
Besides adequate sterilization, the main challenge to using RTU is getting the sealed containers recontamination-free into the grade A core of the isolator or restricted access barrier system (RABS) for filling/finishing.
Two different approaches have been successfully established in the industry:
- No-touch transfer (NTT): Stepwise unwrapping/debagging in higher-quality cleanroom grades, either automatic or semi-automatic debagging; and for NTT, single and double-bagged tubs are established in the market.
- Debagging the closed tub and following re-disinfection of the tub by one of the following techniques:
- Electron beam irradiation
- Chemical disinfection (commonly by hydrogen peroxide (H2O2) fog/vapor
- Pulsed white light with ultraviolet (UV)
These techniques can achieve safe transfers (there are references to approved production lines within the industry).
Regulatory Framework
Annex 1 and other guidelines do not specifically address transfer of RTU tub systems into grade A 1. However, statements of these guides also apply for RTU transfer or can be interpreted as being applicable. Here are some popular citations:
The FDA’s 2004 Guidance for Industry 2establishes transfers into the aseptic zone as a critical process: “It is critical to adequately control material (e.g., in-process supplies, equipment, utensils) as it transfers from lesser to higher classified clean areas to prevent the influx of contaminants.” However, this guidance focuses more on the number of transfers and the rapid transfer port technology. When this guidance was published in 2004, nested RTU pack styles were relatively new in the market and not yet widely used.
The description of a nest transfer from an article on the history of prefilled syringes in 2015 3says: “Ready-to-use syringes are nested in tubs that are sealed and surrounded by at least two polymeric bags. The bags surrounding the tubs of syringes are removed progressively as the tubs are transferred to the more controlled areas of the manufacturing suites. The first bag may be removed in the grade C (ISO 7) area and then placed in a transition zone where the second bag is removed before transferring the sealed tub into the grade B (ISO 5) area. Finally, an operator will place the sealed tub in the grade A (ISO 5) area and the Tyvek seal will be removed either manually or by the equipment automatically.”
The process described was manual because automatic and robust bag removal systems were rare and double bags couldn’t be peeled automatically at all, so the first removal had to be done manually. This situation created the need for safer transfers with basically no manual intervention. Sections of Annex 1, with relevance for the transfer of RTU pack styles should be considered 1:
Section 4.10
This section gives a general heads-up to the process risk of any transfer in and out of grade A environments. This applies to all kinds of goods to be transferred regardless of their transport packaging. This includes equipment such as tools and tweezers, components such as stoppers, and pre-sterilized pack styles of vials and syringes.
Section 4.11
This section states that any transfer be performed in a unidirectional process, ideally with a direct interface between a sterilizer and grade A (“double door autoclave or depyrogenation oven/tunnel”) 1. This is not possible for RTU because RTU material rarely gets autoclaved. Ethylene oxide or gamma irradiation are the sterilization processes of choice for RTU with gamma for plastic containers only.
These processes must be performed off-site in dedicated facilities. RTU material is then transported in multiple shells (i.e., tub, inner bag, outer bag, liner, or carton) to the manufacturing site. Once there, it must undergo a safe transfer procedure for introduction into grade A fill/finish.
Section 4.12
This section asks for protection of materials transiting through grade B (“Equipment and materials (intended for use in grade A area) should be protected when transiting through grade B area”) 1. This protection is provided for RTU pack styles, which come in (multiple) wraps to the aseptic manufacturing line. The concept of this section is based on a core aseptic processing cell in grade A quality, which is surrounded, or at least supplemented with a grade B infeed. The ideal “onion skin” concept features a transfer from grade D (or clean nonclassified area [CNC]) to a grade C classified area 1. From there, the material should pass through grade B (“transit”) and be introduced to grade A 1.
This concept can be varied with additional measures. Annex 1 states that the minimum required cleanroom grade for an open isolator environment is grade C (Section 4.20, among others) 1. The transfers into and out of an isolator do not pass a grade B. This, however, is fine as long as differential pressure and unidirectional airflow (UDAF) of grade A air provide a barrier against recontamination. The transferred goods must be sterile.
Section 4.18
This section covers barrier technologies like isolators and RABS: “The hazards introduced from entry or removal of items during processing should be minimized and supported by high capability transfer technologies or validated systems that robustly prevent contamination and are appropriate for the respective technology 1.” However, it remains unclear what features and controls are expected to make a specific technology highly capable for transfer. The Barrier Technologies chapter does not include more details for transfer” 1. However, the chapter “Aseptic Preparation and Processing” in Section 8.10, Table 4, guides typical examples of operation in different cleanroom grades 1.
The description of operation examples in grade A “Aseptic filling, sealing of containers such as ampoules, vial closure, transfer of open or partially stoppered vials” does not describe the typical situation for introducing packed/wrapped RTU containers 1. This is covered more under grade B examples: “Conveying or staging, while protected from the surrounding environment, of equipment, components and ancillary items for introduction into grade A 1.”
The examples list is incomplete and does not give guidance specifically for RTU pack style transfers. It reflects more a static exposure of a protected item before a transfer process (“conveying or staging”) and not necessarily the transfer situation itself 1.
Section 8.46
This section is part of the sterilization chapter and addresses the RTU situation from the sterility point of view: How to maintain sterility during storage and transfer 1. Multiple sterile coverings are listed and a stepwise removal during transfer into grade A is described: “…configuration of the sterile pack allows the items to be readily disinfected during transfer by operators into grade A (e.g., by use of multiple sterile coverings that can be removed at each transfer from lower to higher grade) 1.” Section 8.46 describes the typical NTT situation with multiple covers.
Section 8.47
This section gets more specific on the transfer technique and gives advice on re-disinfection: “…this should be done using appropriate validated methods (for example, airlocks or pass-through hatches) with accompanying disinfection of the exterior of the sealed packaging 1.” The concept behind this is that the bioburden on the outer covering of a sealed and pre-sterilized package will be removed by disinfection during the transfer step. This is an obvious requirement for keeping grade A free of microbiological contaminants. However, it does not necessarily apply to the RTU situation with multiple coverings (this is covered in Section 8.46).
The validation requirements on the packaging of the presterilized RTU tub systems is addressed in Section 8.48 stating that the RTU user validate the full supply chain process, from sealing and sterilizing at the RTU supplier to transfer into grade A. Besides thorough validation, Section 8.48 also demands a check prior to use: “…the integrity of the sterile protective barrier system for each of the sterilized items should be checked prior to use 1.” In case of RTU kits, this check is commonly performed visually.
Both approaches (NTT and re-disinfection) are valid and could be linked to Annex 1 without being directly addressed by it (creating uncertainty and misleading interpretations). Consequently, this paper examines the details of RTU transfer solutions from the perspective of quality risk management, demonstrating that there exists a multitude of technologies for safe transfers.
Continuous learning from production data and quality assessments in a defi ned risk review process helps keep risk management updated.
Quality Risk-Based Approach
The availability of high-quality medicines is a key intention of Annex 1. In the dualism of quality and availability of medicines, a balance of measures must be taken to the benefit of patients. The tool set for this is a holistic quality risk management process according to ICH Q9 (R1) 4. It starts with a risk assessment, which consists of hazard identification, risk analysis, and risk evaluation. After risk assessment, the process adds appropriate and effective risk control measures.
The third element of risk management includes risk communication, which helps to install an orchestrated mitigation process and keeps team members informed. Continuous learning from production data and quality assessments in a defined risk review process helps keep risk management updated. This is documented and kept up to date in the contamination control strategy (CCS), according to Annex 1 1.
Risk Assessment
The primary risk during RTU transfer is product contamination by germs, originating from any point in the supply chain. Contamination could occur at the RTU manufacturing stage (despite validation and controls), during transportation to the fill/finish facility, during warehousing or unpacking, or within the transfer section itself, potentially leading to adverse patient reactions.
In the first approximation any kind of contamination (viable or nonviable particulate), the contamination is taken as a hazard, which should be addressed. Risks associated with the hazard could come from the following:
- Contaminated surfaces in the transfer section and unbagging equipment
- Operator interventions (with open doors or through glove ports)
- Insufficient separation of the processing area from the operator’s environment (through barrier or damaged HEPA filters)
- Contamination carried into the product flow during the material feed of the debagging station
The probability of potential risks depends very much on the configuration of the equipment and handling practice of the operators, which must be addressed in a thorough process design. In a robustly automated debagging and transfer process, risks deriving from operator interaction are limited to routine handling of package material (material feed at debagging station or, in case of double-bag transfer, at the first debagging station) and manual handling of environmental monitoring goods through glove ports.
Risks coming from unplanned operator interventions (e.g., with open-door access of the transfer section barrier to fix a given problem) are very critical. These risks increase the likelihood of recontamination of the inner bag and, in consequence, recontamination of the tub. This also impacts the risk severity, as particulate might be transferred from the operator. NTT should be “no touch” to avoid indirect contamination routes.
The risk analysis should also cover the second debagging step and the situation with the inner bag open and tub transfer into the grade A aseptic core zone. Even if recontamination of the inner bag should have happened (via whatever contamination route), contamination spreading to the sealed tub and transferring into the grade A zone should be avoided.
Risk Mitigation Measures
The first measure to reduce recontamination risks is to apply a cleanroom approach in the transfer section, comprising a barrier enclosure, a pressure concept with higher pressure level within the transfer section (compared to the environment), HEPA filtration of incoming air, and UDAF. This is combined with a pre-cleaning and pre-disinfection step before production starts.
Additional measures needed for operating a grade B or grade A environment include the control of the cleanroom quality by sensors (e.g., differential pressure levels upstream and downstream terminal air filters), particle counting, active viable sampling, and settle plates. Whatever grade is claimed for transfer arrangement is a result of a thorough risk analysis and must be reflected in the CCS with an appropriate process design, including technical and operational measures. The same applies to the inactivation efficiency when a re-disinfection process step is part of the transfer.
Re-Disinfection
Electron Beam Treatment
The first representatives of re-decontamination systems were electron beam systems (see Figure 1), which provide electron irradiation to re-disinfect the surface of the tub 5, 6. The irradiation dose of at least 15 kilo-Gray (kGy) is a validated industrial standard to inactivate six logs of any kind of microorganism on the outside of the tub and at locations just below the Tyvek cover sheet 7. There are many established systems that provide a 25 kGy dose (equivalent to a 12-logs reduction of bacillus pumilus spores). These bring a higher assurance level but increase exposure of RTU containers to oxidative side products of the process.
The inactivation dose must be achieved under the nonwoven cover because the cover is not flush in shape with the sealed area (overhang), and therefore the gripping tab corner could potentially, but very unlikely, be contaminated. Due to this penetration of the nonwoven lid, electron beams could hit the glass containers and result in discoloration. Therefore, RTU manufacturers deliver specific electron beam packs with an extra (double) sheet of Tyvek inlay in the tub to decrease the dose at the surface of the glass containers (see Figure 2) 8. Tubs for electron beam re-decontamination are commonly wrapped in a single (outer) bag because potentially transferred contamination during bag opening would be inactivated anyway.
Side Effects
A side effect of electron beam irradiation at atmospheric pressure is the generation of nitrogen oxides and ozone in the irradiation zone, which must be exhausted quantitatively. Residual concentrations of these gases are harmful to operators and products. Because the glass containers are protected by the double Tyvek liner, there is no dose at the container level; therefore, suppliers can also demonstrate no ozone at container level. Electron irradiation also generates some X-ray emissions, which must be blocked by geometrical measures (a labyrinth-like arrangement of the parts flow in the electron beam tunnel) and armor plating of the tunnel with lead sandwich composites.
Electron Beam Design
Well-designed electron beam tunnels have grade A in the transient zone between electron beam curtain (re-decontamination) and isolator infeed. This requires UDAF airflow and pre-disinfection, commonly performed with an H2O2 bio-decontamination process before production starts. Additionally, a pressure difference between isolator (e.g., + 25 pascals [Pa] to grade C isolator environment) and re-decontamination zone (e.g., – 20 Pa to grade C electron beam environment) generates a strong counterflow of grade A air. This flushes the re-decontaminated tub on its way to the isolator. Generally, the transient zone is particle controlled using HEPA filters and, as an option, they can be equipped with particle counters. Viable monitoring depends on electron beam type: the accessibility to exchange nutrient media might be limited. Well-designed systems offer remote viable sampling points.
Electron beams need lead shielding to protect operators from X-ray exposition. This results in a significant weight of the systems, which must be considered for the facility design (maximum load on the floor per square meter). However, commonly the electron beam systems are very compact and space saving in design. Validation of an electron beam process is straightforward: color strip dosimetry is commonly applied to determine the dose distribution on the tub surfaces.
Alternative technologies were explored to address the recontamination risk by tub transport, storage, and handling of an already sterile (ex-works) tub. Chemical re-disinfection or an alternative physical disinfection process, such as UV or pulsed white light with UV shares of spectrum, was investigated as alternatives to electron beam and NTT.
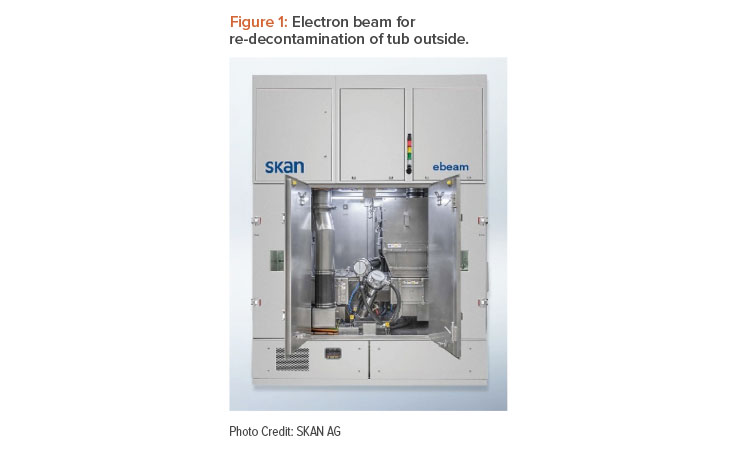
Pulsed Light
UV is a clean and simple technology with a simple dose control capability. It treats the outside only (no dose absorption inside the tub) and creates little or no relevant oxidative atmosphere in the treatment chamber. This could deteriorate materials or generate persistent residues that might harm the product 9, 10. With the pulsed light approach, flashes (e.g., 300 milliseconds) transfer enough energy for many types of microorganisms to cause cell layers to burst and initiate DNA decomposition. But some pigmented germs (like aspergillus brasiliensis) might be persistent.
The disadvantage of UV is the low penetration capability. Nontransparent layers shield germs from interaction with UV irradiation. For example, the nonwoven cover sheet cannot be penetrated and so the gripping tab corner of the tub will not be treated. Multilayer stacks of microbiological contaminants will also not be completely inactivated, as the residues on the top layers might shield the bottom layers from UV energy (i.e., shadow effect).
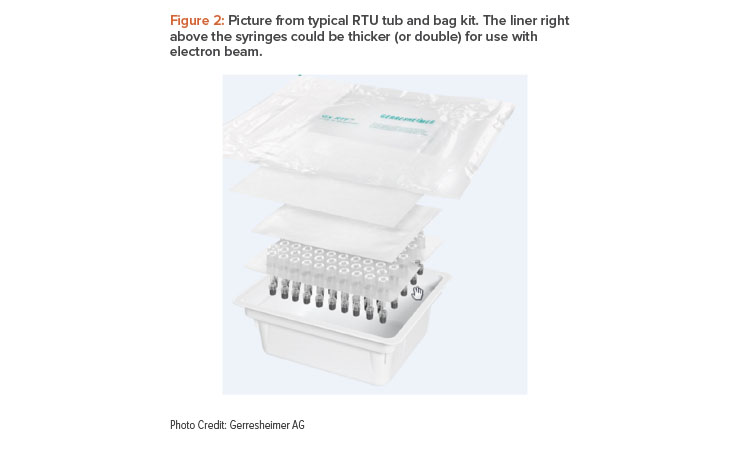
Chemical Agents
A chemical bio-decontamination agent must get into contact with all tub surfaces. The contact time must be short to achieve a high output (e.g., six tubs per minute) and residuals of the disinfection reagent must be aerated within the shortest time to avoid contamination or deterioration of the product.
A common chemical for re-disinfection is vapor-phase hydrogen peroxide (VPHP)11. The vapor has the capability to penetrate the nonwoven cover sheet and thereby provides decontamination of the gripping tab corner. However, hydrogen peroxide will also migrate into the tub and interact with the packaging material. For glass-only containers, this is not an issue because hydrogen peroxide will be aerated/diluted as soon as the tub is opened and exposed to UDAF within a short time. With rubber and polymer components (e.g., tip caps), however, this might take much more time. For oxidation-sensitive products like proteins, a risk analysis backed by data must show that peroxide residuals stay below a concentration level which might harm the product quality.
Vaporized hydrogen peroxide is the decontamination chemical applied to RTU tubs in a recently introduced continuous transfer system vapor-phase hydrogen peroxide 12. In this setup, the contact with the hydrogen peroxide vapor is only seconds long. Therefore, a high vapor concentration (6.000–8.000 parts per million [ppm]) is provided to the outer surfaces of the tub. Up to six tubs per minute are claimed as maximum decontamination and transfer speed. The tubs must be unbagged before introduction into the bio-decontamination tunnel. This could be done with an automatic or semi-automatic debagging machine.
The bio-decontaminated tubs are aerated by HEPA-filtered UDAF with their nonwoven lid still sealed. The second step of aeration happens as soon as the lid is peeled off in the fill/finish isolator with grade A airflow (UDAF). Compared with the low-output setup, this system has the same advantages and disadvantages: effective disinfection of the tub surfaces (6 log, also in the gripper tab area), but penetration into the tub, which leads to residuals that must be removed before filling oxidation-sensitive products. As the tubs are moved in the tunnel during the bio-decontamination process, it is not possible for any part of the tubs to avoid contact with the chemical disinfectant.
Risk Control: NTT
NTT concepts rely on a robust process design, which starts with safeguarding the sterility of the bag inside, preventing recontamination during unwrapping/transfer, and removing particles before the tub enters the tub opening and filling section. Because there is no re-disinfection step involved, each step must be well controlled and should be automated for reproducibility and separation from operator.
In the case of double-bag systems, the concept for folding the inner bag varies with packaging material vendor. An automatic unfolding/opening process must be able to flexibly handle whatever tub/bag configuration comes on the line. However, this flexibility might result in rare handling errors, which could be addressed with an intervention for keeping the product flow running 13.
Intervention could principally mean robotic intervention (no need for operator access), manual intervention through glove ports (doors stay closed), or manual open-door intervention. This last option should be avoided wherever possible. Depending on the type and frequency of interventions, different cleanroom requirements and routines are needed.
Avoiding jams by robust debagging technology is a major driver for risk reduction. This includes sourcing the highest quality packaging material with a reproducible folding of the inner bag. In case of stops created during the unfolding step, transport or debagging, the next line of defense is to prevent particulates from being transferred during the following intervention from the operator or the operator’s environment to the process.
For NTT, an open-door intervention is clearly the riskiest measure to fix a problem. But depending on the problem and the ergonomic situation, it could also be the last resort to resume production. Interventions through glove ports are significantly reducing the risk, particularly when performed with sterilized tools.
After intervention, the cleanroom (and surface) quality of the transfer section must be recovered to validated levels. This might include sporicidal treatment and dwell time on all surfaces in and close to the intervention area. Packaging material, which has had contact with gloves or tools during intervention, needs to be removed and should not be further processed.
Following the path of potential contamination, “blockage – open door intervention – transfer of contaminant,” the last line of defense is the airflow design at the debagging and transfer station. As long as the inner bag is closed, the residual risk of contamination transfer to the tub and via the tub into the grade A zone is very low/negligible. In the moment the bag is opened with a knife, the tub should not have any contact with the inner bag around the open bag “mouth.” This is also important for the following pushover of the tub into the aseptic core zone.
Double-Bag Systems
A basic requirement for NTT is a robust bag opening and tub transfer system. Double-bagged RTU packages are becoming more popular due to double safety against damage and support of a stepwise transfer into higher-grade cleanroom environments. In the past, bag opening of double bags was commonly a manual process because the inner bag is folded around the tub and must be expanded before an automatic opening process of the inner bag can be started. Otherwise, the knife would not completely open the bag and, consequently, the tub could not be transferred without additional manual cutting intervention.
Meanwhile, different automatic technologies in the market are available to expand the bag without manual steps, which reflects Section 2.1.i. of Annex 1: “…the use of appropriate technologies… should be considered to increase the protection of the product from extraneous sources of endotoxin/pyrogen, particulate and microbial contamination… 1.” Some technical solutions are listed next.
![Figure 3: Vacuum chamber (labeled #32) for unfolding the inner bag [14].](/sites/default/files/pe/2025/Jan-Feb/0125_PE_JF_Raus_03.jpg)
![Figure 4: Retraction (labeled #68) mechanism [15].](/sites/default/files/pe/2025/Jan-Feb/0125_PE_JF_Raus_04.jpg)
![Figure 5: Spiking mechanism (labeled #40) [16].](/sites/default/files/pe/2025/Jan-Feb/0125_PE_JF_Raus_05.jpg)
![Figure 6: Bristle rollers (labeled #12a and #12b) [17].](/sites/default/files/pe/2025/Jan-Feb/0125_PE_JF_Raus_06.jpg)
Vacuum Chamber
The vacuum process starts with the transfer of the tub into a vacuum chamber. Doors are closed and pressure is lowered, causing the bag to expand due to the pressure difference between the inner bag and the chamber. After aeration of the chamber, the bag presents an easy-to-cut flap on the side of the tub. After releasing the tub from the vacuum chamber (double door load lock style), it can be transferred to the bag cut and removal machine (see Figure 3) 14.
Grab and Retract
In an alternative process, the tub with the inner bag is presented to roller arrangement, which grabs the plastic film and unfolds the flap by a movement against the transport direction. The package is retracted, which stretches the flap to be cut. From there, the tub with bag can be transferred to the cutting and bag removal station (see Figure 4) 15.
Pressurization by Spiking
The application of a pressure difference between the inside of the inner bag and the environment is the basic principle of another solution for unfolding and grabbing the flap for the bag opening process. In this concept, a puncture with a hypodermic pressurized needle into a piece of plastic film of the inner bag, which is grabbed by a suction dome principle, creates an overpressure in relation to the environment. This helps inflate the bag and allows the flap to be unfolded and cut. This process can be combined with a vacuum dome to increase the pressure difference (see Figure 5) 16.
Grab with Bristles
Instead of inflating the inner bag, this solution uses the friction between the plastic film and a set of rollers with bristles to grab the folded flap of the inner bag. The two rollers are positioned one above the other and rotate against each other to pull the flap between them 17for stretching and cutting (see Figure 6).
Tub Opening and Filling in RABS
A very common expectation is to do transfers from CNC to D, to C, to B, to A (or vice versa). In the case of a RABS line, the aseptic core (grade A) has a grade B environment. For unplanned interventions and routine manual operations (e.g., change of settle plates or opening of rapid transfer ports), gloves are required. In exceptional cases, the doors might be opened, but this is a very critical process and it must be based on a detailed description in the CCS and properly documented. Partial line clearance together with re-disinfection of the area of intervention might be an appropriate measure to get back into production. As with other equipment design, the door opening is combined with the end of batch.
NTT with Double-Bagged RTU Packs
Although NTT with double-bagged RTU packs in RABS lines is not common, it should still be noted. There are two different cleanroom settings possible for transfer into RABS lines with NTT and double-bagged RTU material. These are a grade B inside transfer section and a grade C inside transfer section.
Grade B Inside Transfer Section
The first bag is removed in a grade C environment and transferred into a grade B environment with the inner bag still closed to prepare for the opening of the inner bag and transfer into the fill/finish core. The transfer section between the bag opening units needs to have glove ports for handling goods, which are needed to perform environmental monitoring (EM) (e.g., plates for active and passive viable monitoring). This is a requirement for claiming grade B.
The same glove ports could be used to fix problems with tub transfer and bag opening processes. The environment of the transfer section could have a grade C classification. In traditional aseptic manufacturing, this would require a pressure difference between the operator environment and the inside of the transfer section of at least 10 Pa, according to paragraph 4.14 of Annex 1.
To achieve this pressure difference, the setup would need a closed RABS-like design. Alternatively, the operator environment is a grade B (same as inside). But this arrangement (grade B in a grade B environment) is rare. It might make interventions easier because under the regime of standard operating procedures (SOPs) doors could be opened to fix jams. Nevertheless, for interventions and exchange of settle plates or active air sampling, gloves would still be preferable.
Because no openly exposed product or unprotected components are handled in the transfer section, a grade B could be achieved (i.e., classification, monitoring) without claiming pressure difference of at least 10 Pa, according to paragraph 4.14 of Annex 1. Airflow visualization should support the separation claim.
Grade C Inside Transfer Section
Because the tub is still sealed and containers are not exposed to the environment, the inside of the transfer section could principally also be classified as a grade C cleanroom with ISO 5 airflow. With the opening of the inner bag, the tub will be flushed with grade A air from the isolator (pressure difference) and filtered ISO 5 airflow from UDAF of the transfer section, as described previously. The risk of recontamination by a lower-grade process environment depends on the process. This is especially true on the airflow design in the opening step of the inner bag before the tub enters the grade A zone of the aseptic core.
The operator environment would be a grade C in this configuration. That is, interventions could be performed via glove ports or under strict adherence to detailed SOPs by door opening. Direct touch of the inner bag should be avoided as sterilized tools would be preferable. The particle status can be monitored to control grade C, but clear separation between the inside of the transfer section and the operator environment is not shown in data (both grade C). It boils down to a holistic risk evaluation of packaging material and transfer and to operator handling to make this simple setup robust.
The doors should stay closed all the time. If there is a need for opening the doors to fix a jam, material disposal, a disinfection program, and further safeguards should be applied to avoid any cross contamination during interventions. These safeguards could include sterile gloves for operators and optionally UDAF above door swings. Airflow visualization studies should support the separation claim.
Open Isolator Tub Opening and Filling
Grade A Inside Transfer Section
The transfer of the tub and opening of the inner bag is handled in an isolator section upstream to the tub opening isolator with an operator environment of grade C. Although there is no installation known to the authors, this is principally possible, but requires a significant additional effort compared with an open RABS. It would be the most secure situation but would come with some technical and handling challenges such as the disposal of the inner bag in a contained setup, e.g., with a rapid transfer waste port.
Also, in this configuration, a pressure difference between the tub opening and the section for opening or removal of the inner bag would make sense to avoid particulate from the bag opening being transferred into the open tub. Therefore, a pressure cascade from the isolator section to the opening section would have to be defined and monitored.
Interventions in the transfer section to correct the position of the tub or assisted unfolding of a sticky/flappy inner bag must be performed through glove ports. Opening the door would result in a major interruption with repetition of the bio-decontamination cycle and additional challenges in the mousehole area. Overlapping bio-decontamination of the seals would hardly be possible.
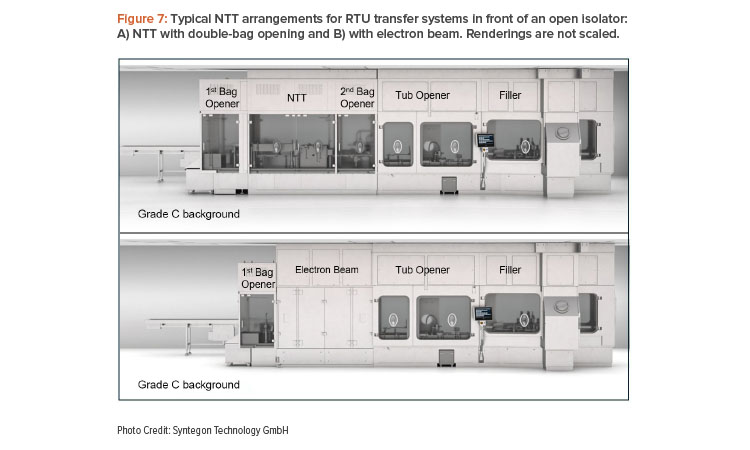
Grade B Inside Transfer Section
As described previously in the RABS section, the first bag is removed in a grade C environment and transferred with the inner bag still closed into a grade B environment to prepare the bag for opening. Grade B requires cleanroom monitoring (e.g., particle counter, active air sampler, settle plates, and monitoring of filter pressure difference). For the exchange of EM media, gloves need to be installed in a RABS-like barrier. The gloves can also be used for manual interventions so that doors can stay closed.
A classified grade B in a grade C environment would require a pressure step between the operator environment and the inside of the transfer section. This could only be achieved by a closed RABS with return air ducts. But the operator environment could principally be either a grade B or a grade C. Grade B would be exotic. This is because in a fill suite layout with an open isolator there is no other grade B area that could be expanded.
Placing the grade B transfer section in a grade C environment without pressure difference from grade C to grade B is combined with a pressure step from the transfer section to the isolator inside (grade A). This results in the overflow of grade A air into the bag opening area and forms an “grade A air jacket” around the tub. The required pressure difference is therefore only moved from grade C/B to grade B/A and combined with automated stepwise removal of covers, as described in section 8.46 of Annex 1 1.
Grade C Inside Transfer Section
This convenient approach uses the isolator in a grade C (operator) environment, which is expanded by the area of the transfer section. Integrating gloves into the doors might address risks from direct operator intervention. The bags are still closed, and tubs are still sealed. Interventions in the opening and unloading process of the inner bag should be prohibited anyway. A major issue in this area would lead to the end of batch. Two examples of typical arrangements for RTU tub transfer into an open isolator are shown in Figure 7. One example is with NTT and one is with re-decontamination represented by an electron beam setup.
Closed Isolator Tub Opening and Filling
As per the definition in the Annex 1 glossary 1, closed isolators do not have a continuous flow of material into and out of the enclosure. In this case, tubs must be transferred and opened internally before fill/finish processes can start. A common approach involves an H2O2 chamber with a mousehole to fill/finish section of the work cell. The tubs must be unbagged manually and loaded into the hydrogen peroxide treatment chamber still sealed with the nonwoven cover. The hydrogen peroxide vapor penetrates the tubs and bio-decontaminates the tub outside with some residual concentration inside.
The chamber for this does not have a defined classification but provides HEPA-filtered air to flush the compartment. After the bio-decontamination process, tub after tub is handed over from a magazine in the bio-decontamination chamber through a passive mousehole into the fill zone. That principle is also an example of “no-touch-transfer” from a nonclassified (but bio-decontaminated) section with HEPA-filtered UDAF into grade A, but this time in a closed setting with no operator access. A closed isolator setup is very much dedicated to small batches because storage volume and handling of nested components (e.g., snap-fit caps) is limited. This setup is also common with fast transfer H2O2 airlocks docked to the isolator or tubs hanging in the isolator during initial isolator bio-decontamination cycle.
Criteria | No-Touch-Transfer | Electron Beam | Pulsed Light | Hydrogen Peroxide |
---|---|---|---|---|
Accessibility of equipment and surfaces to cleaning and sanitization | Cleaning and disinfection procedures for different machines to be established and validated | Manual cleaning of the inner chamber, automated bio-decontamination process (H₂O₂) for the last chamber | Automated bio-decontamination process for the pulsed light chamber | Automated bio-decontamination process for the H₂O₂ chamber/tunnel |
Material flow | Tub commonly in double bag in grade C | Tub at least in single bag in grade C | Tub at least in single bag in grade C | Tub at least in single bag in grade C |
Need for interventions by personnel | Glove interventions required if bags are folded incorrectly | Robust transport of tubs into class A without intervention | Robust transport of tubs into class A | Robust transport of tubs into class A |
Frequency of interventions | Medium | Low | Low | Low |
Complexity of process | Unwrapping/debagging in front of e-beam: cleanliness classes: grade C / unwrapping outer bag → irradiation of outer tub surface → grade A / tub opening | Unwrapping/debagging in front of pulsed light chamber: cleanliness classes: grade C / unwrapping bag → decontamination with pulsed light → grade A / tub opening | Unwrapping/debagging in front of H₂O₂-decontamination chamber: cleanliness classes: grade C / unwrapping bag → decontamination with H₂O₂ → chamber grade A / tub opening | |
Infrastructure requirement | High: space requirement for different machines: a) manual, semi-automated, or fully automated outer bag opening station b) automated inner tub opening, lid removal | Weight of electron beam needs appropriate building structure: a) manual, semi-automated, or fully automated bag opening b) automated tub opening, lid removal | Low (chamber), Medium (tunnel): a) manual, semi-automated, or fully automated bag opening b) automated tub opening, lid removal | Space requirements for tunnel: a) manual, semi-automated, or fully automated bag opening b) automated tub opening, lid removal |
Microbiological reduction | No reduction | Decontamination (6 log at 15 kGy or sterilization (12 log at 25 kGy)) | Disinfection (reduction 4 - 6 log) - as per manufacturer's information | Decontamination (reduction 6 log) |
Impact to product | No impact | No or low residuals of nitrogen oxides and ozone | Little or no oxidative atmosphere | Low H₂O₂ residuals in glass vials < 50 ppb |
Complexity of validation | Intensive airflow visualization study at mousehole to grade A - EM in transfer section | Color strip dosimetry to be applied - Residuals study required for sensitive products | Validation of microbial reduction using biological indicators - Residuals study required for sensitive products | Validation of microbial reduction using biological indicators - Residuals study required for sensitive products |
Health authorities acceptance | Accepted, but different interpretations of measures | Accepted | Not yet fully established | Accepted (H₂O₂ process for decontamination) |
A safe transfer process with the appropriate technology will minimize risk. This is done with attention to every process step after sterilization of the packaging material at the supplier.
Aerodynamic Measures with NTT
Airflow studies have been performed to show the aerodynamic situation in the last debagging and transfer step 18, 19. One of these studies was published before Annex 1, in 2022. The other study was published in 2024. In the last debagging step, manual interventions should be avoided. However, in case unplanned interventions are necessary, they should follow the same SOPs known from interventions in the fill/finish environment with openly exposed aseptic products. The goal is to avoid any transfer of potential contaminants (e.g., particles, microbes) from the bags or the bag opening environment to the tub.
The article “No-Touch Transfer (NTT)” 19demonstrates some of the difficulties in designing the airflow. Vertical UDAF meets horizontal overflow from the isolator (triggered by the pressure difference between the isolator and the debagging environment). Cutting equipment, flaps of the inner bag, and the tub front face present a barrier to the horizontal airflow, which leads to deflection. This could create a turbulent situation that might be limited by a thorough airflow design. Factors that could impact airflow include the velocity 20and direction of the airflow coming from the HEPA filters above the debagger and leaving to the return air duct openings in isolators, airflow opening grids in a RABS, or the pressure difference between grade A (isolator) and grade B or C (bag opener).
Risk Communication and Review
A safe transfer process with the appropriate technology will minimize risk. This is done with attention to every process step after sterilization of the packaging material at the supplier. These steps include incoming goods control, warehouse storage, cleaning and disinfection of debagging and transfer equipment, maintenance of automation and cleanroom technology, robust recipe parameters, and trained interventions, if needed.
According to Annex 1, the central document for risk analysis and mitigation is the CCS 1. It is the guide through the whole process for production management, operators, quality department, and authorities. A CCS is a living document with regular reviews 21. It serves to document the decisions taken. Regular reviews will include results from continuous process verification and new measures taken as a result of effectiveness checks.
Conclusion
In this article, the different systems for RTU components have been described with technical details, advantages, and disadvantages. All systems described are in use in industry and have proven to be effective. It is up to the user to evaluate them for individual applications based on a sound risk assessment as documented in the CCS.
Table 1 gives an overview of NTT and re-decontamination processes that could be applied to RTU (tub system) transfers. The criteria listed address important aspects for decisions, but the list is far from covering all aspects of process design and capex criteria.
As already mentioned, the new Annex 1 strives for quality and availability of sterile medicinal products. In choosing cleanroom settings and transfer processes, microbiological aspects are only one side of the decision framework. Technical complexity and robustness of equipment, availability of skilled personnel, facility constraints, and affordability of solutions are further criteria to be evaluated for a well-considered decision.