Measuring Pharma’s Adoption of Industry 4.0

With the rise of new technologies and predictive analytics capable of handling the huge amounts of data within and across existing information systems, Industry 4.0 has been thriving in many sectors, such as industrial automation, financial technology, retail, and semiconductors. But the health sector in general,
Given the significant advances digital technologies have brought to the automotive, agricultural, and retail industries,
Pharmaceutical regulations have expanded globally since the early 1960s, leading some observers to suggest that resources dedicated to meeting regulatory requirements and exhaustive quality control are diverted from R&D and innovation.
Furthermore, health authorities are making efforts to spur innovation in the pharmaceutical industry. For example, in 2017, the US FDA created the Emerging Technology Program,
Industry 4.0 Research
In 2017, Liao and colleagues completed a systematic literature review of the past, present, and future of Industry 4.0,
Some examples of technologies that have seen early adoption in industries other than the pharmaceutical industry include infrared spectroscopy,
The Influence of Regulators
Regulatory agencies can influence the implementation of innovation, as illustrated by the thalidomide episode and the American pharmaceutical industry. After the incident, an attributable slowdown was confirmed by the American pharmaceutical industry, and there was widespread impact that lasted for more than 10 years after the fatal episode.
However, regulators work to support innovation: Initiatives promoted by public administrations like the Emerging Technology Program
Pharma 4.0™
During the opening plenary session at the 2018 ISPE Continuous Manufacturing Workshop,
Determining the pace of adoption for specific technologies in the pharmaceutical sector and other manufacturing industries can provide a clear picture about the overall pace of adopting innovation.
Pace of Adopting Innovation
Determining the pace of adoption for specific technologies in the pharmaceutical sector and other manufacturing industries can provide a clear picture about the overall pace of adopting innovation and, specifically, the lack of innovation attempted by pharmaceutical manufacturing. Drugs are products with sensitive impact in society, and they need to be well controlled throughout their entire life cycle. Taking this assumption as a legal imperative, regulators have a crucial role around the entire process. In terms of innovation, regulatory agencies must determine how new technology implementations will impact the quality, safety, or efficacy of the final product and ensure these technologies are under control and can be implemented without risk to the patient.
Comparative Study
In this comparative study across industries, historic moments will be examined, including when specific technologies were adopted by pharmaceutical companies compared with early adopters in other industries. Looking at similar technologies that have been adopted by the pharmaceutical industry and comparing their early acceptance with when they were endorsed by other sectors can help illustrate the different pace of technology adoption between both groups and provide a clear picture about the lag experienced by pharmaceutical manufacturers. (Actually, the pharmaceutical industry is still progressing through the automation challenges related to Industry 3.0, although it may bypass further implementation and progress directly to Pharma 4.0™
This idea can be extended to other functions within the pharmaceutical industry, specifically the large difference between R&D and manufacturing.
For example, the ICH Q8 guideline referenced previously was designed to help companies, reviewers, and inspectors perform their tasks more efficiently. The foundation of this guidance establishes the demonstration of deep knowledge of pharmaceutical and manufacturing sciences as the main driver to create a basis for flexible regulatory models. An explicit reference to near-infrared (NIR) is designated in this document as valid technology for real-time release when it is properly described in terms of process understanding within the submission. The guidance describes how the implementation of NIR for unit dose uniformity control would be integrated into the process when the expected uniformity is achieved, without waiting for a fixed time as is usually required in classic recipes. But NIR is not the only spectroscopical technology used to perform homogeneity tests; other implementations include Raman or mass spectroscopy. When more than one technique is available for a specific innovation, those techniques are also considered for inclusion in the documentation.
The selected technologies have been identified as relevant and innovative applications that were deployed in drug manufacturing at some stage of the product life cycle. To be included in this study, the technologies had to be referenced by regulatory bodies. Records associated with official regulations in the pharmaceutical industry supporting or describing guidelines for the proposed technologies have been included in the analysis to identify potential links among official constraints and speed of technological adoption effects.
Technologies as Transformation Enablers
The collected records correspond to technologies that are considered transformation enablers within manufacturing processes because the industries experienced improvement after or during implementation of these technologies. Examples of enhancements in the pharmaceutical industry provided by innovation are associated with a reduction of variations brought on by the inevitable manual operations and uncontrolled properties of raw materials. Applying this reasoning, the following technologies were considered in the research.
Spectroscopy
Spectroscopy allows real-time testing during manufacturing. It is usually mentioned in pharmaceutical guidelines motivating systems for nonintrusive measurements and is broadly referenced for PAT applications.
Chromatography
Chromatography is a multivariate technique used for substance identification in production environments and for purification in biotechnology processes. It is used to separate components in mixtures, presenting different methods depending on the characteristics of the components contained in the sample. Using chromatography for purification is an implementation widely applied in pharma-biotechnology operations, and it is well established in the pharmaceutical and other industries.
Lyophilization
Lyophilization is a physical process in which water is eliminated by sublimation in products and the manufactured item is then subjected to vacuum conditions. Lyophilization is extensively used in the food industry and mainly applied in drug manufacturing for batch process freeze-drying and continuous process spray-drying.
Radio Frequency Identification
RFID is a widespread technology used in logistics and manufacturing to track and ensure traceability of materials and products, and it requires sophisticated mechanisms that have been globally adopted. RFID enables total traceability along the product supply chain. Product traceability is required in drug manufacturing and one of its most known applications is to avoid counterfeits.
Artificial Intelligence
Although is a computing term that, strictly speaking, is not a technology, the introduction of this discipline in the industry boosted technological breakthroughs driven by the Industry 4.0 wave,
3D Printing
3D printing is a technology born in the 1980s that creates three-dimensional objects by adding layers of material to fill sequential and consecutive sections of the object. More than 30,000 patents regarding 3D printing have been reported in the US alone, and its industrial expansion is mainly due to support from open-source computer programs.
Innovation | Discovery or Invention | Nonpharma Industry | Pharmaceutical Industry |
Regulatory |
---|---|---|---|---|
NIR Spectroscopy | 1800 |
1938 |
1977 |
2004 |
Mass Spectrometry | 1917 |
1920 |
1990 |
2003 |
Raman Spectroscopy | 1928 |
1987 |
2002 |
2004 |
High-Performance Liquid Chromatography (HPLC) |
1941 |
1966 |
1972 |
1994 |
Gas Chromatography | 1952 |
1962 |
1985 |
1994 |
Lyophilization | 1890 |
1935 |
1950 |
1993 |
Radio Frequency Identification (RFID) | 1948 |
1973 |
2005 |
2007 |
Artificial Intelligence (AI) | 1956 |
1988 |
2012 |
2017 |
3D Printing | 1984 |
1986 |
2009 |
2015 |
Big Data | 1985 |
2002 |
2011 |
2019 |
Big Data
Big data is computer science technology in which huge volumes of data belonging to a large variety of records are accessed at high velocity, which establishes the main dimensions that characterize a data management system initially architected on the Map Reduce mechanism.
Key Benchmarks in Adopting Innovation
For each technology, four dates were extracted from the literature: year of discovery or invention, year first used in the industry, year first used in the pharmaceutical industry, and year it was considered by regulatory bodies. Table 1 contains the raw dates and the references from which they were extracted.
Figure 1 shows the adoption lead times of the selected technologies: first, the date of discovery or initial use, followed by adoption by nonpharmaceutical industries and then pharmaceutical industries, and finally inclusion in regulatory references. Although the periods vary depending on the technology, there is a consistent delay for all technologies regarding their adoption in the pharmaceutical industry, indicating later endorsement of the innovations in drug manufacturing and inclusion in regulations as well (taking the first implementation in other sectors as reference). The technologies have been grouped by field: chromatography, Industry 4.0 (AI, big data, 3D printing), lyophilization, and spectroscopy.
To establish indicators that may reveal inferences between the pharma industry and industry in general, regulations and gaps between the considered dates are used. Therefore, the following factors will be calculated, all measured in years:
- The time between adoption of these technologies by the pharma and nonpharmaceutical industries (GapPharmaNonPharma)
- The time between the pharmaceutical industry adoption and the first regulatory reference to a specific innovation (GapRegulationPharma)
- The time between invention of discovery of the technology and its adoption by nonpharmaceutical industries (NonPharmaIndustryAdoption)
- The time between invention of discovery of the technology and its adoption by the pharmaceutical industry (PharmaIndustryAdoption)
- The time between invention of discovery of the technology and the first regulatory reference to a specific innovation (RegulationAdoption)
These factors normalize the measurements. The statistics calculated for each, represented by the box plots in Figure 2, indicate the presence of a clear outlier. Applying the Dixon test for outliers over the values corresponding with the discovery/invention date (displayed as Discovery.Initial.use in the box-plot of the Figure 2), produces a p value = 0.087 for the hypothesis that the NIR’s invention date is an outlier.
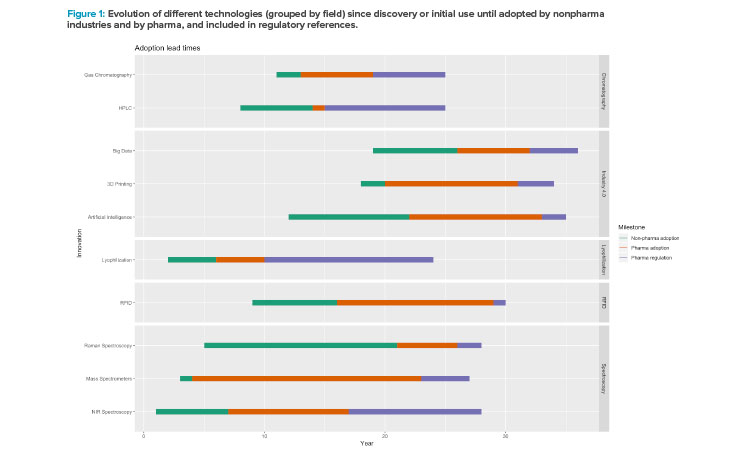
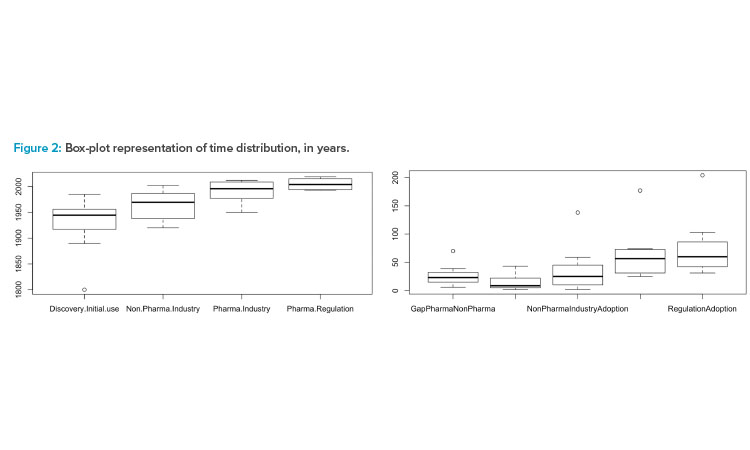
In Figure 2 are Dates (left) when technologies were (a) discovered/invented (Discovery.Initial.Use); (b) adopted in pharmaceutical industries (Non.Pharma.Industry); (c) adopted in biotechnology/pharmaceutical industries (Pharma.Industry); and (d) referenced by regulatory bodies (Pharma.Regulation). On the right in Figure 2 are differences in technology adoption between (a) pharmaceutical and nonpharmaceutical industries (Gap-PharmaNonPharma); (b) pharmaceutical industry adoption and first regulatory reference (GapRegulationPharma); (c) technology discovery and nonpharmaceutical industry adoption (NonPharmaIndustryAdoption); (d) technology discovery and pharmaceutical industry adoption (PharmaIndustryAdoption); and (e) technology discovery and regulatory reference (RegulationAdoption).
Due to this evidence, the outlier is removed from the data set.
Considering this configuration, the results indicate that although there are records referencing the initial usage of technologies in the industry generally 24 years after their discovery or invention, on average the pharmaceutical industry assimilates initial usage 48 years later (±6 years). Furthermore, technology implementation by early pharmaceutical adopters was led mainly in R&D, not in manufacturing. Regulatory bodies included the sampled technologies an average of 12 years after they were implemented in pharmaceutical activities. Beyond this figure, a reaction can be observed in the agencies when drug companies prove the feasibility of innovative systems in their internal structures. Two indexes can be created for comparing the pharmaceutical innovative adoption:
The measurement of the pharmaceutical early adoption (PEA) provides the ratio among the pharmaceutical field and the industry implementation of a specific innovation. The smaller the index, the faster the innovative adoption is in the pharmaceutical industry compared with other industries. Values for PEA greater than 1 indicate a slower process of incorporating innovation in the pharmaceutical industry. For the set of technologies discussed in this approach and represented in the Table 1, the value of PEA = 1.99.
\(\text{PEA=}\frac{PharmaIndustryAdoption}{ NonPharmaIndustryAdoption} = 1.99 \)
Comparing how quickly regulation includes new technologies compared to companies, the ratio among the difference of time needed by regulatory agencies and pharmaceutical companies and the time required for companies introducing these technologies, gives a measure of the innovative attitude of the administrations. This coefficient can be identified as REA (regulatory early adoption) and is calculated taking the pharmaceutical company as reference and not the industry because for the regulatory bodies, the implementation of new technologies only make sense once they have been accepted by pharmaceutical companies. For the set of technologies considered here, REA = 0.28, which means that the regulatory bodies are faster than pharmaceutical companies in integrating innovative applications inside their mechanisms of control.
\(\text{REA=}\frac{GapRegulationPharma}{ PharmaIndustryAdoption} = 0.28 \)
Pharma’s Delay
Sorting the technologies by their invention date and observing the adoption speed by nonpharmaceutical industries (using the NonPharma Indus-tryAdoption factor) and by pharma (using the PharmaIndustryAdoption factor), a systematic delay is observed along the history experimented by the drug manufacturing. Furthermore, the trend evidenced in Figure 3, confirmed by both categories (pharmaceutical and nonpharmaceutical industries), can be interpreted as an acceleration in the adoption of new technologies in more recent decades.
Values in Years | Mean* | Standard Deviation | Mean Variation |
---|---|---|---|
Gap Pharma-NonPharma | 25.60 | 18.54 | 5.86 |
Gap Regulation-Pharma | 13.70 | 13.20 | 4.17 |
Nonpharma Industry Adoption | 24.22 | 19.04 | 6.35 |
Pharma Industry Adoption | 48.33 | 19.73 | 6.58 |
Regulation Adoption | 60.55 | 24.16 | 8.05 |
* The mean variation ( (𝞂𝞂/√N) ) is calculated considering N including the outlier associated
to the NIR technology only for the Gap Pharma-NonPharma and Gap Regulation-Pharma
variables.
Creating sets of technologies which cluster similar innovations or keeping the specific invention as a standalone, there are five groups that can be compared by means of the PEA and REA indicators to measure the differences between the pharmaceutical industry and regulatory bodies in terms of the innovative attitude.
The spectroscopy group contains the NIR, Raman, and mass spectroscopy techniques; the chromatography group includes the HPLC and the gas systems; the Industry 4.0 group includes AI, big data, and 3D printing; lyophilization and RFID technologies constitute two independent measures that cannot be aggregated with any other innovation. The results of calculating the PEA and the REA values, based on the average of the elements for each category or using the individual values for the classes with only one element, are shown in Table 3.
Conclusions
The pharmaceutical industry can be deemed a key sector in the industrialized world for several reasons. From an innovation perspective, drug production is recognized as an industry that invests huge amounts of economic resources in R&D, where technology is a main constituent in development strategies. On average, 10% of sales is invested in the area that establishes the approach and deployment of sophisticated systems, aiming to control the production process and the facilities.
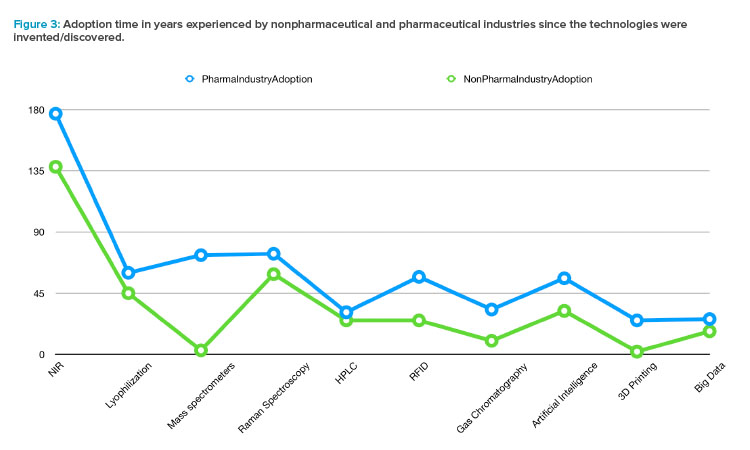
Spectroscopy | Chromatography | Lyophilization | RFID | Industry 4.0 | |
---|---|---|---|---|---|
PEA | 1.62 | 1.83 | 1.33 | 2.28 | 2.10 |
REA | 0.13 | 0.48 | 0.72 | 0.04 | 0.18 |
Finally, drugs have been designed to provide positive outcomes for patients and to improve the welfare of the population, even though there is an undeniable risk of unfortunate episodes (where people died or the treatment did not provide the expected outcome). Although some recent opinions regarding a relaxing attitude in regulatory agencies could be misunderstood,
From a patient-centric perspective, the slower innovative attitude in drug manufacturing in comparison to other industries (see Figure 3) is justified by the required control of the fabrication process to preserve the safety of patients. As regulatory agencies have repeatedly shown, they act quickly in front of critical episodes such as the coronavirus pandemic. The innovation delay cannot be explained by regulatory obstructions.
On the other hand, the introduction of new technologies in compliant guidance has been always faster than their implementation in the pharmaceutical industry, as can be observed by the REA and PEA factors in the Table 3.
Notice that the innovations linked to equipment requiring physical contact with drugs during the manufacturing operations (spectroscopy, chromatography, and lyophilization) present a PEA lower than those technologies not used to directly manage the product. However, the regulatory agencies are slower to include those techniques in their guidance than innovations not physically in contact with the drug (for example, big data or AI). The 3D printing technology is an exception in the Industry 4.0 group (PEA = 12.5, REA = 0.24), which could be related to the intrinsic relationship between the physical process creating drugs by this technology and the Industry 4.0 basis.
Finally, an acceleration can be ascertained from the regulatory agencies, including technologies developed under the umbrella of the Industry 4.0 (REA = 0.18), whereas pharmaceutical manufacturing is keeping a similar pace (PEA = 2.10) than observed for other innovations.
To ensure more rapid adoption of novel technologies, we recommend the pharmaceutical industry work more closely with all the actors involved in the community, including regulators and suppliers, to speed up the regulatory framework of these technologies, as the pharmaceutical industry can play a critical role in this process.
Acknowledgments
A technical review of this document was performed by Andrej Ondracka, who provided valuable feedback in the format and content of the document.