Biotech Industry’s Quest for Optimized Manufacturing Facility Design

Since the early 1990s, when the “upstart” biotech industry realized that its future success would be heavily influenced by the ability to manufacture multiple products within the same facility,
In Search of Flexibility: The Biotech Industry’s Continuing Quest for Optimized Manufacturing Facility Design
A Brief History of Biotech Facility Design
The first edition of the ISPE Biomanufacturing Facility Baseline Guide,2 published in 2004, included a graphic (Figure 1) that painted a well-defined picture of how facility design evolution had been influenced by a combination of regulatory guidance shifts, equipment technology advancement, and manufacturing operational approach changes. The main driver of this evolution was a developing, clearer understanding of the impact of closed-system design, as was defined in the Baseline Guide.
Figure 1 illustrates the significant impact that the shift from open-system operations to a more closed-system approach (where validated closed-system design in manufacturing equipment allowed for a loosening of area classification requirements) had on facility design and the ability to provide more flexibility in manufacturing options, especially for large-scale monoclonal antibody (mAb)–focused products.
As shown in cases 1–4 in Figure 1 from the ISPE Biotech Manufacturing Facilities Baseline Guide and in the case 2 example in Figure 2, moving from the “traditional” extensive open-system classified space to a more closed-system design implementation yielded operational and maintenance cost savings as well as schedule flexibility and improvement.2
The Arrival of Single-Use Systems
In the mid-2000s, the impact of single-use technology and its advancement in equipment design launched a shift in how the biotech industry viewed facility design, which influenced the operational approach for many companies. As acceptance from global regulatory agencies for single-use system (SUS) implementation grew, companies shifted the design approach to incorporate both the closed system and single-use system attributes into solutions that focused on footprint reduction, greater layout flexibility, and reduced operational costs (Figure 3).3
Closed Systems and Controlled Nonclassified Space
Addressing the industry need to better define operational characterization around closed systems, the BioPhorum Operations Group (BPOG) focused on addressing flexibility in biomanufacturing operations; the impact of closed systems served as a driver to implement an operational philosophy around controlled nonclassified space. Their 2011 publication was the first challenge to current industry thinking around the need for classified space to support biologic drug substance manufacturing, and it opened the door for new views on flexibility.4
With BioPhorum Operations Group’s challenge to the facility design and operational paradigm came the next step in the design approach shift: the introduction of the open “ballroom.” The early concept of a ballroom approach was driven around the principle that validated that closed, single-use, or hybrid systems could be operated within a single manufacturing space (Figure 4) and not increase product contamination risk.5 Because physical segregation was the most expensive solution to protect the product, this approach received tremendous interest from both industry and regulators.
The publication of the BioPhorum Operations Group ballroom approach6 gave credibility to the idea of flexible manufacturing for biologic drug substance in a batch-driven approach. The first major manufacturing facility to implement the ballroom approach into biologics manufacturing elements and receive licensure from the FDA was the Amgen Singapore Biologics Manufacturing Facility. This facility (Figure 5) received significant attention in the industry due to its central manufacturing suite concept, where a large number of unit operations are executed in an “open” ballroom suite.7
The key benefits of this type of approach include projected lower cost per gram of produced protein, increased flexibility for future site implementation, and capital cost savings of hundreds of millions of dollars due to 80% reduction in size.8
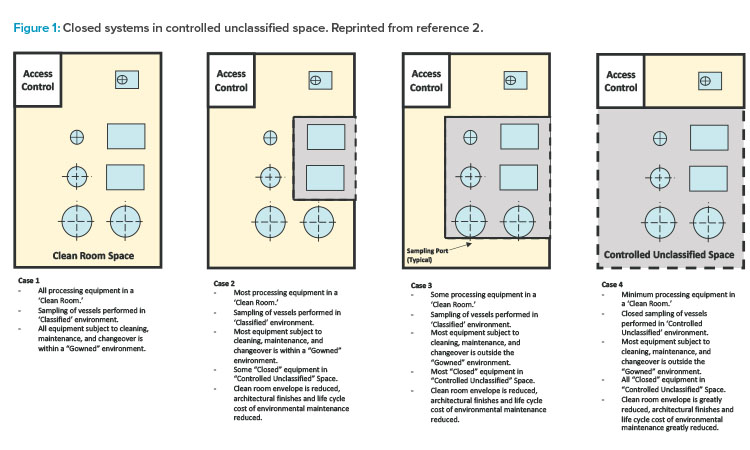
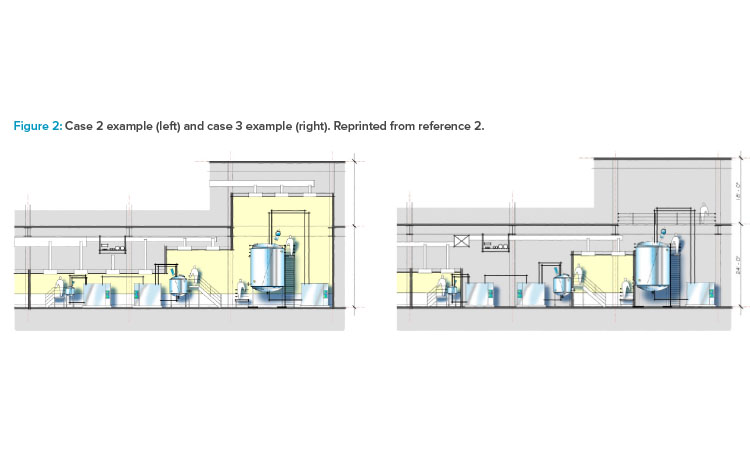
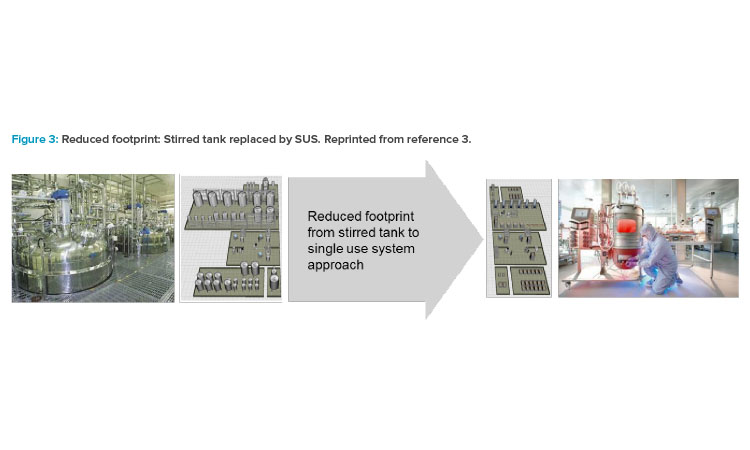
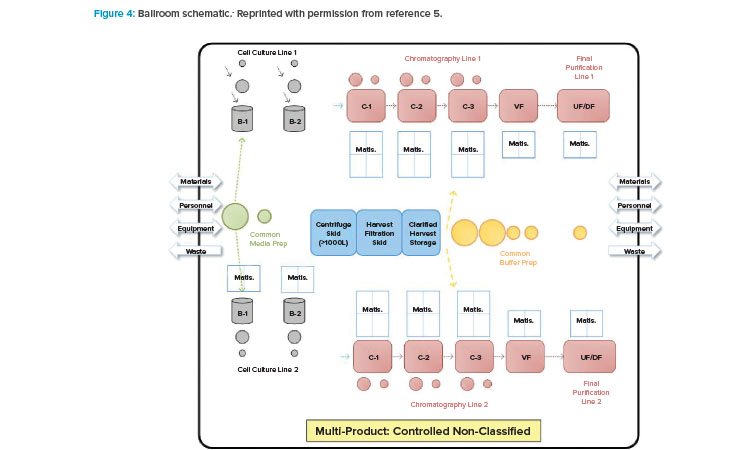
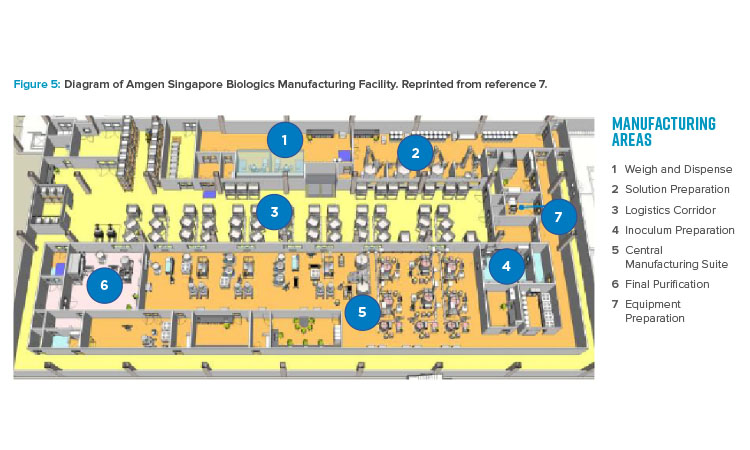
Current Trends
In the 2015 BioPlan Associates Top Trends in Biopharmaceutical Manufacturing,9 three of the listed trends were the implementation of single-use system , flexible facilities driven by modularity, and continuous biomanufacturing. The following discussion explains how these three facility design drivers, as well as robotics, continue to shape biomanufacturing optimization efforts.
Single-Use Systems
As early as 2006, single-use system implementation began to impact facility design and offer flexible solutions.10 Today, single-use system implementation, coupled with proof of closure, has become a key driver of process and facility design that drives flexible solutions.11 The impact of single-use system implementation and the advancement of its regulatory acceptance to support product protection and reduce patient risk have been the focus of numerous industry forums and conferences.
A key focus of the second edition of the ISPE Biopharmaceutical Manufacturing Facilities Baseline Guide12 was to better define current design trends driven by flexibility. The revised Baseline Guide not only established the foundational relationship between facility attributes and system closure but also, for the first time, addressed the relationship between risk assessment, process closure, and layout approach.
Modularity
Standardization, rapid deployment, and reduced schedule execution are all attributes of the current drivers to investigate modular delivery platforms for manufacturing assets. Over the years, modular approaches, or modules, have been defined from process skids (late 1990s), to prefabricated building segments (late 1990s), to “super-skids” of major equipment and piping components, to the current prefabricated modular cleanrooms. Today, much of the focus is on modular approaches that use forms of prefabricated panel systems or modules that allow for rapid deployment.
The combination of single-use system and modularity is driving the next generation of flexible solutions that are primarily being offered for operations focused in the under-2,000-liter-scale operating range for a wide range of product types, including advanced therapeutic medicinal products (ATMPs).
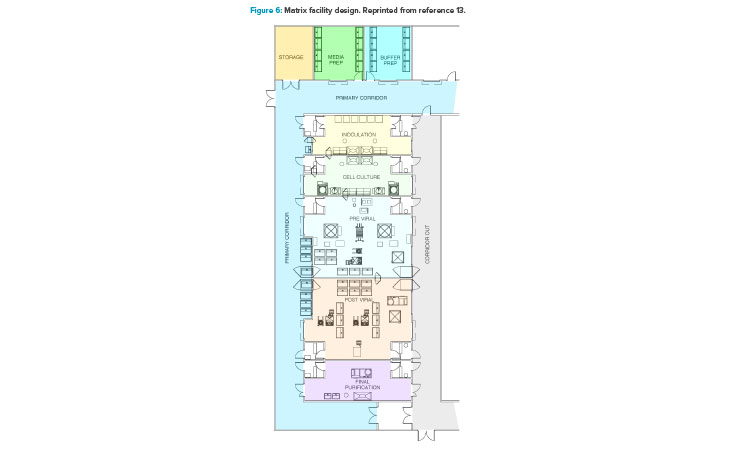
In addition to the ballroom approach already presented, another facility design trend to promote optimized operations is a more segregated layout configuration (Figure 6).13 This segregated “matrix” of suites allows for tremendous flexibility between upstream and downstream operations, campaign and concurrent manufacturing, and the ability to conduct short (clinical) and long (launch) campaigns within the same facility.13 This design approach accommodates both small- and larger-scale manufacturing operations with high levels of flexibility and reconfiguration capability. It also comes with a higher capital cost element due to the increased physical segregation of both environmental and architectural elements.
Continuous Manufacturing
Continuous manufacturing will increase focus on facility design flexibility. The ongoing movement to advance and incorporate continuous manufacturing into the biotech industry is supported by the FDA,14 which in turn is driving the industry to focus on how the advantages of continuous manufacturing should be defined in facility design attributes. Some of the keys requirements include:
- Smaller equipment and facilities, which would lead to lower capital and operational costs and the potential for modular units
- Increased flexibility in operations
- Integrated manufacturing, which would allow fewer processing steps and simplification in scale-up
These drivers all are in synergy with the pursuit of optimized manufacturing, again being supported by the FDA.15 But challenges remain as continuous manufacturing technology in biomanufacturing develops. First, this technology is difficult to implement for some unit operations, and a “hybrid” process design could result. Second, there are regulatory concerns around risk—can closed systems be implemented 100%? And, finally, the long history and investment in batch-processing influences the overall design approach.
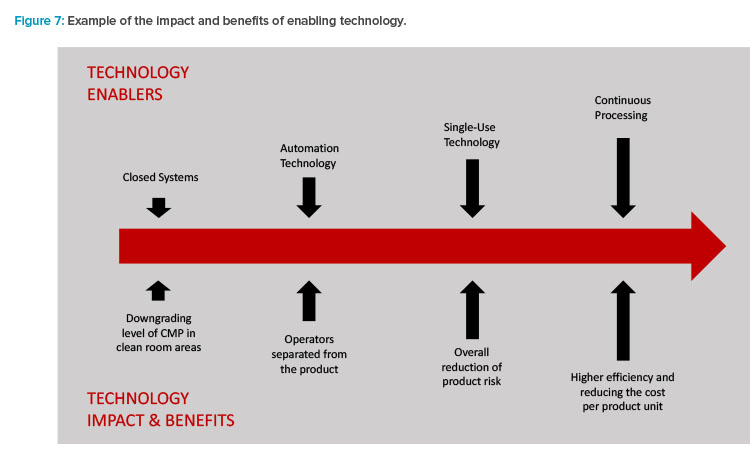
It is important to understand that implementation of continuous manufacturing is not a given, and it is not the right solution for every company. Because of this, the Parenteral Drug Association (PDA) developed a guided decision process to answer the question of whether to implement single-use system, and that process has often been adapted for continuous platforms.16 For organizations today, continuous manufacturing is not a one-size-fits-all solution.
Robotics
An estimated 80% of errors in pharmaceutical production are due to human error.17 Risk reduction is a priority in process and facility design today. As higher-level automation solutions move into the use of robotics for drug product and drug substance manufacturing, the industry is taking robotic technology to the manufacturing floor as a major aspect of the Pharma 4.0TM movement.
Current applications include material handling and transfer such as buffer replenishment; compounding activities for toxic materials used in anti-cancer drugs; picking for kits (syringes, vials, needles, etc.), sampling, packaging, and labeling; and numerous applications in aseptic fill/finish processes.
The combination of these current trends now reflects the mission set forth in the first part of to this article. Enabling technologies (Figure 7) are driving the results and benefits that the industry needs and desires.
Personalized Medicine: The Final Frontier?
The increased focus on ATMPs is shifting the flexible facility paradigm even more. As far back as 2011, the industry recognized that future facility design was going to need to move into new directions.18 The current baseline model defining the majority of biomanufacturing operations for human therapeutics (proteins) is batch-driven. Because advanced therapies target either specific groups of patients or individual patients (personalized medicine), efficient commercial production will not be achieved with the large process volumes and higher titers of traditional biopharmaceutical manufacturing assets. This scale of manufacture and the 1:1 treatment-to-patient nature of autologous therapies mimic hospital lab or compounding pharmacy operations, but the need to produce these therapies for larger patient populations in a safe, pure, and effective manner will require GMP-regulated facilities.
One of the key challenges as ATMPs move along the continuum from development to commercialization is how to ensure these products will meet current GMP guidelines, regardless of whether they are being developed in an academic or commercial environment. All the design and regulatory at-tributes and trends that have been previously discussed come into play for these facility types.17
Today, ATMP manufacturing facility design represents the blurring of traditional lines that the industry previously established. These facilities enhance the need for flexible solutions, enabling technologies, and out-of-the-box project delivery: the “perfect storm” of facility optimization. Small-scale, rapid timeline development and execution, and technology innovation will be the attributes driving optimized facility design.
Future State
Moving into the next decade, key questions are: Does the enhanced focus on flexibility and optimization in biomanufacturing point to a new paradigm model? Will the future state eliminate our decades-old approach of facility design for many of the new biologic therapeutics?
Large-scale, stirred tank-based, mAb-focused manufacturing assets will be needed for the foreseeable future. They will likely remain a workhorse of the industry and continue to hold a significant place in the manufacturing landscape due to both investment and robust results. But how will the future state look for small-scale single-use platform (<2,000 liter) ATMP-focused manufacturing needs?
Two manufacturing operation models may provide a glimpse into the future state of biomanufacturing. The first is an adaptive approach to contract manufacture in a “one-stop-shop” solution, where equipment suppliers drive innovation and process development solutions targeted at specific client product-process-facility attributes of the enterprise.
Modular,single-use system-focused process solutions will be designed around centralized manufacturing support and logistics needs, and will be leased, similar to current contract manufacturing organization–type business models. The flexibility aspect will include in-house supply of raw materials, media and buffer components, QC support, filling and packaging, warehousing, and distribution.
The second model scenario takes the form of modular, flexible GMP units that can be either individually or collectively used to support small-scale ATMP-focused operations. Because of an increasing interest in decentralizing manufacturing operations for smaller-scale operations, these units (which can be rapidly deployed and easily configured) will be developed around specific equipment platforms and can be located at hospitals, research facilities, or commercial-focused incubators. This approach will address concerns over meeting facility-driven GMP requirements and will rely on a GMP-focused operational approach for validated equipment, systems, and facilities.
Conclusion
The search for optimized biomanufacturing facility design led us far from where we started in the 1980s. Our future path looks very different as well. We seem to be moving toward smaller facilities with higher output capabilities. The need for lower capital cost requirements remains, along with an increased focus on reduced time-to-deploy delivery models with significant flexibility due to single-use system technology. Smaller, faster, and less expensive are the new “normal” for many organizations.
The capital demands on manufacturing organizations continue to be an area of focus to reduce costs. Capital expenditures for manufacturing assets have a direct tie to the cost of goods and the overall financial health of an organization. Today, many companies face tough decisions due to aging facility assets, changing technology demands, increased pressure on speed to market, and the need for agility and flexibility. The traditional path of “build your own asset” is also being challenged with new approaches to manufacturing capability.
Equipment suppliers will continue to move further into the reference frame once solely occupied by design consultancy firms to deliver one-stop-shop facility solutions. This movement is being fueled in part through the numerous marketing authorization application activities in recent months involving organizations such as Thermo Fisher and Pall. The development-to-patient supply chain model may also no longer be just the landscape of traditional biologics manufacturers. Patient-specific commercial manufacturing models will come out of the shadows to occupy a greater piece of the manufacturing landscape. Global regulators define manufacturing control guidelines, and both academic and patient care institutions will become elements of the manufacturing supply chain.
We have come a long way in four decades, and only time will tell where we will be at the end of this decade. However, it is becoming clear that old ways of thinking around facility design and operation will be pushed to the limit.