Environmental Sustainability in Biopharmaceutical Facility Design
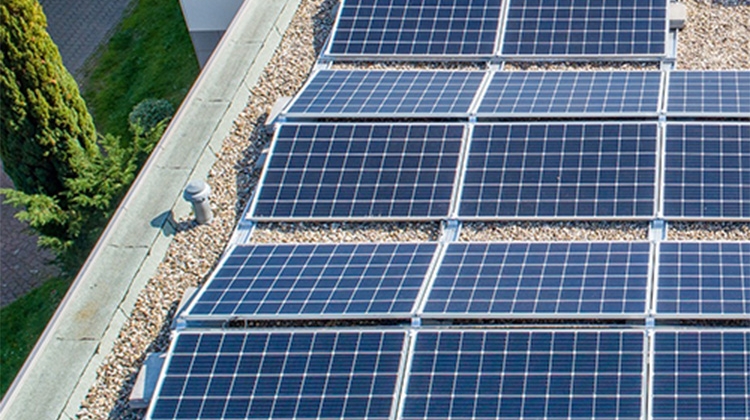
Many emerging tools and technologies support the environmental sustainability of the pharmaceutical industry. In facility design, solutions are derived through science-based analyses of environmental impacts from the materials, processes, services, and architecture. From greener energy sources to a reduction in the type and amount of emissions, we are seeing improvement in this sector’s environmental footprint.
While the term sustainability encompasses a wide scope of goals, in examining manufacturing environmental sustainability we address reducing environmental burdens and progressing to more circular economies throughout the product and manufacturing process life cycle.1 This view prioritizes steps that reduce the use of natural resources and the release of undesirable products such as carbon to the atmosphere or plastics to landfills.
Sustainability Tools and Design Processes
When promoting of an initiative’s environmental sustainability ES, we should include a more comprehensive context that considers planet (natural capital), people (social capital), and profit (economic capital).2 To establish the most relevant facility design criteria against this triple-bottom-line concept, some have defined such categories as a company’s ESG (environmental, social, and governance) performance.3 The pharmaceutical industry, while providing life-saving and empowering services to the world, recognizes the UN’s recommendation that every sector contribute to comprehensive environmental sustainability, included in COP27.4
The biopharmaceutical industry is taking steps to increase its environmental sustainability,5 which begins with identifying and testing alternatives to existing procurement, materials, logistics, equipment, services, manufacturing processes, packaging, and facility design6 (see Table 1).
Sustainable Design Tool | Example Activity | Potential Value |
---|---|---|
Geographical Placement | Locating facility near green energy or abundant water sources | Reduction of carbon emissions and precious water demand |
Building Type | Reusing an existing facility or commercial geographical setting | Reduction of construction materials usage and urban sprawl |
Suite Classification | Using closed, automated systems and nested higher classification | Reduction of HVAC supplies and energy in highly classified suites |
Digital Transformation | Enabling optimized design and intensified processing operations | Creation of more product per suite footprint, materials, or energy |
Processes | Improved resource management: materials, products, operations, personnel, and production schedule | Optimization of energy usage timing, net energy demand, and product batches per facility and time |
Operations Waste | Mechanical, chemical, or energy recycling for single-use plastics | Reduction of land, water, and atmospheric pollution |
Transportation/Logistics | Considering material, final product, and personnel transportation, e.g., packaging, distance, and mode | Reduction of carbon and contaminated water emissions and secondary packaging waste burdens |
Process Equipment | Demand optimization, double com-missioning, and facility electrification | Reduction of current or future demand for fossil energy sources |
Energy Sources | Using local wind, photovoltaic, geoexchange, and heat pump tools | Reduction of fossil fuel consumption or scarce municipal green-grid energy use |
Services | Using systems that reduce net water demand and purity classifications | Reduction in potable and energy-demanding water for injection (WFI) use |
Design Tools | Using modern BIM/CAD architectural modeling tools to supply 3D–7D facility modeling capability | Optimization of suite volume, classification, energy use, HVAC, and classified suite footprint |
Building Materials | Using slag, ash, post-consumer glass and rubber, and existing concrete for construction aggregate | Reduction of energy-consuming aggregate generation and transport; reuse of post-consumer waste |
Durable Facilities | Designing for flexible, modular, future-proofed production suites | Reduction of rebuild and remodeling activities decreases emissions |
Emerging Information | Conclusions from WHO, UN, COP27, Environmental Protection UK, or EPA | Better understanding of the problems and best approaches to solutions |
Green Building Certification | Conclusions from assessment and ratings organizations will identify green status and key directions | Revelation of sustainable solutions for human health, compliance, public relations, and financial benefits |
Financial Considerations | Using PACE loans; federal, state, and utility incentives, foundation grants; and win/win synergistic solutions | Discovering that green process and facility design does not necessarily result in incremental capex/opex costs |
Ranking these individual burdensome activities can be influenced by local goals from corporate commitments, customer expectations, regulatory agencies such as the Environmental Protection UK or EPA, organizations such as the WHO and UN, recognized accreditation/certification organizations, the press, and employees, as well as regional and national laws and codes.7
An integrative (vs. linear) design process is central to successfully attaining such comprehensive sustainability goals and project needs (see Figure 1). This involves not only considering architecture, engineering, and construction professionals, but also encouraging early and global collaboration of all stakeholders and building users in addressing the sponsor’s aspirations. This team’s goal is to identify environmentally sustainable solutions that are integrative and even synergistic.
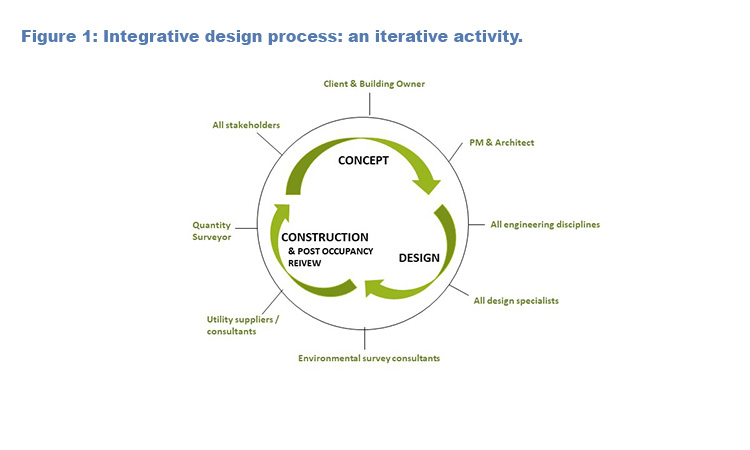
Labeling and Organization
There are various ways to group the many types of environmental sustainability challenges and goals. They follow different criteria, such as the environmental burden’s nature, physical location, or activity generating the effect (see Table 2).
Theme of Organization | Example Domains of Categories |
---|---|
Location of an environmental burden | The earth, natural resources, human health |
Midpoint category burden grouping | Ecosystem, global warming, eutrophication |
Location of burden’s consequences | Atmospheric, terrestrial, hydrological |
Business component causing burden | Facility design, operations, logistics |
Operational activity causing burden | Materials, process, facility service |
Greenhouse Gas Protocol Initiative | Gas sources from Scopes 1, 2, and 3 |
However, they must be categorize to efficiently consider activities topically and in outline. Yet, as potential environmental sustainability solutions are identified, one should consider any tradeoffs or synergies to other systems or categories and prioritize the comprehensive, net results. This is important for two reasons: 1) all actions have secondary environmental, social, economic, ergonomic, and efficiency consequences, and 2) because sustainable facility design is a relatively new and evolving field there are many nonintuitive considerations.
Developing a hierarchy of environmental design criteria in the context of other project goals becomes practical in deciding upon solution options. Systems thinking can help identify comprehensive impacts and outcomes, and many now employ more Pharma 5.0 and mathematical modeling in such systems initiatives.8 Finally, appropriate environmental sustainability solutions are relative to each business− there is no one-size-fits-all and a life-cost analysis, i.e., to evaluate the economic performance of a building or process over its entire life can be very helpfull.
Certification Systems
Green building rating/certification systems such as by LEED and BREEAM help define, measure, and compare the sustainability of facilities. For example, the LEED rating system groups remediation activities within such overall categories as Energy and Atmosphere, with credits or points aggregated into general levels of certification, such as silver or gold. Building or project types are differentiated into such categories as new build or major renovation (LEED BD+C) or operations and maintenance (LEED O+M).
The LEED system is iterative and flexible, and there are many opportunities to reduce energy consumption and improve efficiencies in the building services. It also provides an opportunity to gain certification points by demonstrating innovative ways of reducing energy use not covered by the named categories.
To demonstrate that a goal or credit is achieved, it must be quantifiable. There are a number of internal and external reasons to establish baselines and benchmarks in addition to rating system support. Beyond aiding in achieving such environmental sustainability compliance objectives as carbon emission reduction, certifications offer public relations, financial, and employee health benefits. For example, they can support the development of tax incentives, grants, features to attract tenants, and increased building value.
ISPE exemplifies this in their Facility of the Year Award (FOYA) to recognize innovation in manufacturing facilities, and frequently awards recognition of the category of Sustainability Excellence.
Science-Based Approaches
The use of science-based approaches such as life-cycle assessment (LCA) methods have become mainstream. They identify the emissions, energy, and activity leading to the environmental impacts of a service, equipment, process, or product. An LCA study involves a thorough inventory of the energy and materials employed through the entire value chain and calculates the corresponding environmental emissions.9 It is powerful in determining the environmental burden of these factors, as well as converting their aggregate midpoint and impact categories into predictions, alternatives, and solutions.
Initial Considerations
Environmental sustainability is a socially contextualized issue and requires diligence to avoid prejudice, preconceptions, assumptions, and common tropes. For example, immediate and visible issues often command disproportionate attention as compared to root causes and the less readily apparent issues. Two important principles here are that 1) there are few perfect and complete solutions: we should therefore strive to improve rather than solve, and 2) little progress can be made unless activities are both funded and actively supported by management.
There is a perception that an environmental sustainability prioritized project costs more to develop or operate than conventional designs, but this has been proven in many cases to be a misconception (e.g., by the US Green Building Council). In fact, initiatives such as bioprocess intensification and flexible facility design can not only reduce operational time and expense, but simultaneously reduce the environmental burdens of the process and building.
At a project’s outset, the budget and program should include the time involved for the integrative design process, and the initial capital site, project, commissioning, and certification expenses. Tools such as formal life-cost analysis can aid in estimating total capital and operational expenses (capex and opex), which can then be considered for sustainable versus conventional technologies.
As efficiencies are made in the building footprint, foundations, and structural design, less building envelope is required. A reduced footprint means a reduced roof area, leading to a reduction in stormwater runoff. Reduced building footprint also leads to increased undeveloped area, allowing more landscaping and more space for solar, geothermal or water heat pump equipment.
Sustainable Design Factors and Tools
New Build vs. Retrofit
Remodeling an existing building can be the most efficient strategy to reduce such environmental burdens as near- and mid-term carbon emission totals. It is also the best way to protect undeveloped land. It has been said “The greenest building erection is the one already built.”10 The development of a greenfield site has significant negative environmental impacts: native wildlife habitats may be destroyed, new parking lots and buildings increase storm water runoff and heat-island effects, and increased urbanization leads to greater congestion and pollution.
Retrofitting an existing building to meet comprehensive high-performance standards uses existing building stock, avoiding the release of greenhouse emissions and gasses during demolition and construction, as well as the environmental burden associated with raw material production and transport. Next to the reuse of an existing facility, an infill site, or a vacant lot in a previously developed area, is the best way to protect undeveloped land. Infrastructure and utilities typically exist and a new building on an infill site could add diversity and other opportunities to an urban area. Retaining, retrofitting, or constructing a facility within an urban or suburban area reduces urban sprawl and offers a multitude of benefits that will positively impact both the neighborhood and building occupants.
Geographical Placement
Environmental sustainability is supported by locating a facility in a climate zone where high power in winter and summer is not needed, as is locating in an area supported by a green power grid, freely available water, and existing utility infrastructure. Proximity to both raw material sources and product markets are a factor, as carbon from extended transport can be a continued source of greenhouse gasses. Low-carbon infrastructures for personnel, materials, and product transportation can reduce operational environmental burdens.
Rather than a centralized manufacturing model, which has such logistical environmental challenges as extended highly controlled shipping, a decentralized model allows for manufacturing in closer proximity to the materials sources and/or point of use.
A significant amount of a facility’s carbon emissions can come from staff commuting. Proximity to mass transit, green vehicle support, cycling and walking routes, carpooling, and the close location of anticipated personnel housing can provide a continued environmental sustainability benefit. Construction, local talent, materials availability, and local building regulations are factors in the addressable type, scale, form, and cost of a project.
Environmental Classification
The required suite environmental classifications are determined by specific pharmaceutical processes and products. Besides various particulate concentration limits, procedures or guidance can define such additional requirements as temperature, humidity, and pressure.
Generally, build and operation costs and the operational environmental footprint, as in from energy consumption, increase proportionally to the classification of a suite. Energy consumption is greatly reduced corresponding to room classification downgrading: Grade B environments require in the range of 50–60 air changes per hour, whereas Grade D environments may be as low as 15–20 air changes per hour. It has been reported that increased energy demand, as compared to nonclassified space, is 680% for Grade D, 830% for Grade C, and 2,300% for Grade B.11
As only one single-stage transition in room classification may occur per airlock, designs requiring higher room classifications require more airlocks, thus increasing overall building footprint. For example, when transitioning from a CNC corridor to a Grade B production suite, a total of three airlocks (CNC to D, D to C, and C to B) for personnel and materials is required, as compared to only a single airlock (CNC to D) for a Grade D production suite.
Additional savings here include reduced cleaning regimes (the type and use frequency of cleaning products have a negative impact on facility emissions and indoor air quality) and reduced gowning requirements (including single-use and reusable equipment) as well as determining actual required air flow rates and setting the air circulation rate (ACR ) based on these data.
Recent developments supporting a reduction of the level or extent of suite classification include automated, closed manufacturing equipment; continuous processing; and technologies enabling the nesting of classified space.12 For example, a filling room for an open process will require a Grade A (ISO 5/5) certification, yet functionally closed bioprocesses require only a Grade D, ISO 8 environment. Biosafety cabinets provide a Grade A (ISO 5/5) local environment for operation of open activities but have a Grade B (ISO 5/7) room requirement.
Grade A (ISO 5/5) isolators provide segregation of product from operator and environment and can maintain aseptic conditions for open operations in a Grade C (ISO 7/8) or sometimes even Grade D (ISO 8/undefined) backgrounds.13
Practical examples of implementing closed-process technology resulting in cleanroom classification reduction are:
- A cell therapy facility in Europe that implemented closed, automated cell therapy processing equipment, resulting in a reduction of cleanroom classification from Grade B to Grade C
- An mRNA facility in the US that implemented closed single-use technology for processing, resulting in a reduction of cleanroom classification from Grade D to Grade C
- A large-scale monoclonal antibody facility in the US that employed fully closed, stainless steel bioreactor process trains in a clean, nonclassified space rather than a classified environment (see Figure 2).
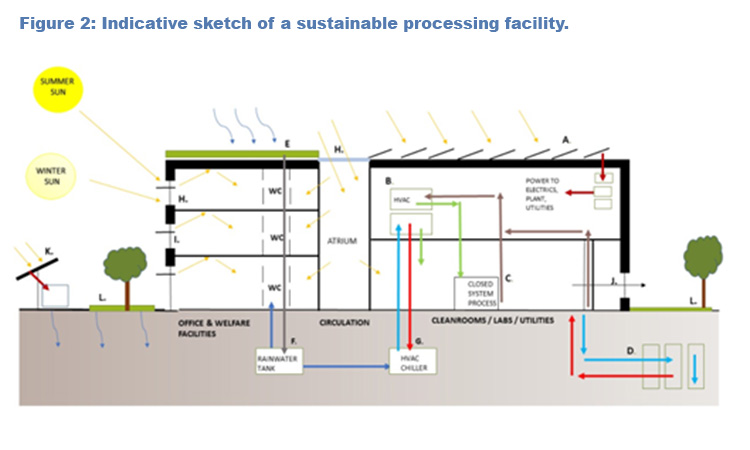
Legend
- Photovoltaic cells
- A highly insulated building with a green roof enables internal temperatures to remain stable
- Using a closed process can reduce the overall cleanroom classification, further reducing the energy burden on the HVAC system: This also leads to a decrease in gowning requirements, single product waste from same, cleaning requirements, and overall facility footprint
- Building up rather than out retains undeveloped open space for things like a geothermal heat pump system
- Green roof insulates the building and retains up to 50% of stormwater runoff usable in many ways
- Rainwater tank
- HVAC chiller using rainwater reduces potable water use
- Windows allowing daylight in, access to natural ventilation (where possible), and views
- Light shelves
- Positioning circulation around the cleanroom allows views outside while reducing risk from direct sunlight/external views into a cleanroom space
- Photovoltaic cell carpark roof/trees
- Landscaping and pervious paving allows direct drainage to the ground
Digital Transformation
The digital transformation of manufacturing includes integrating such digitalized or SMART (specific, measurable, achievable, relevant, and time-bound) advances in supply chain and operations as paperless recording, heightened connectivity, machine language and vision, improved monitoring and analytics, and new process simulation, modeling, and automation. Following inspiration from such initiatives as PAT and Pharma 5.0, many facilities are becoming more intelligent and automated.14
Digitally driven activities that increase the efficiency of manufacturing range from robotization of warehouses and advanced process control to closed, single-use cell processing and automated high-speed filling.15 Further advances and new applications are occurring in such distinct arenas as data science, cloud and edge processing, machine learning and applications, novel and soft sensing, digital twins, drones, modular autonomous skids, and cobots.16
Processes
Many current initiatives for the optimization of manufacturing processes coincidently reduce environmental burdens. Process intensification activities improve the productivity of operations by such metrics as product per cell, time, volume, footprint, or materials. Intensification technologies in biomanufacturing include advanced cell engineering and cloning; inoculation-based shortened process trains, improvements in cell culture media and feeds; dynamic perfusion empowered culture; continuous processing; simulated moving bed, AEX and HIC chromatography; and model-based improvements in active process control.17
Improving production planning and resource management optimizes facility and service utilization and overall energy consumption. Tools such as finite capacity scheduling (FCS) are appearing that consider the real-world capacity constraints of an organization and its enterprise/manufacturing resource planning (ERP/MRP) system, including equipment, tools, labor, and material. FCS modeling accurately predicts operation start and finish times, providing visibility into how to best manage the tradeoffs involved in efficient plant operation. Capacity analysis and activity distribution systems (such as in clean in place and utilities) can also be modeled to support minimizing downtime, optimizing energy usage timing, and reducing net energy demand.
Process Materials
An LCA reveals the overall environmental burden from each material or supplier and aids in understanding their absolute and relative contributions. Life-cycle costing is also important to determine the cost and environmental burden of a product or system over its lifetime — including payback periods, operation, maintenance, replacement, and end-of-life disposal and recycling costs. Optimizing processes to use more environmentally sustainable materials and to reduce materials consumption, expiries, operational waste, and failed batches are valuable in identifying methods treatment or recycling of waste. The importance of understanding environmental burdens from material sources is exemplified by the UK’s Health and Care Act 2022 that will establish a net zero National Health Service.18
Beyond the nominal reasons for its adoption, single-use materials can provide benefits over comparable stainless steel equipment from an environmental sustainability perspective. Examples of this include significant chemical and water use reduction, as well as energy savings from eliminating required cleaning and steam sanitization cycles. However, concerns remain regarding the increasing volume of single-use consumables that are sent to landfills or incinerated.
Considering this, biopharmaceutical sponsors have established aggressive environmental sustainability goals for not only their products, but also their manufacturing processes. Recycling programs for spent single-use consumables include partnerships such as MilliporeSigma/Triumvirate.19, 20
Further advances in recycling technology address the challenges of biopharmaceutical disposables, including the redesign of packaging, the difficulty of recycling materials composed of several different types of plastic, and concerns regarding the safe recycling of contaminated waste.21, 22
In this context, the term recycle has a few definitions. The first issue is, because truly circular economies have not yet arrived, what options that are available can be regarded as “recycling”. Relevant concepts to consider include a) reuse, up-cycle, and down-cycle; b) mechanical recycling (e.g., polymers of ethylene); chemical recycling (e.g., plastic monomers and CH4); and elemental recycling (e.g., C, H, and Cl); and c) energy recycling or recovery (e.g., incineration with profitable heat use).
There have been significant proximate accomplishments in this arena by biopharma sponsors, materials suppliers, and such pharmaceutically oriented public and private organizations as the ACS GCI Pharmaceutical Roundtable and the BPSA23 the Vinal Institute24 and the European Green Deal25 in proposing new EU-wide rules on packaging.
Process Equipment
Closed and automated equipment is becoming popular for a variety of reasons, such as economy, efficiency, and enhanced product safety. However, it can also reduce the environmental burden of a process. For example, it supports the reduction of the suite classification and energy-intensive personnel demands. Benefits of modular, flexible, and skid-based equipment include the long-term benefit of supporting efficient changeover between processes and products. It also provides savings in extensive installation and validation activities during scheduled changes, as well as futureproofing against required facility alterations.
Electrically driven equipment is often more energy efficient than conventional, has lower maintenance costs, and, in some cases, has lower BTU/kWh demand. If the current grid is not green, electrification of a facility will nevertheless result in the potential to exploit greener power as the grid improves. Providing charging stations for personnel electric vehicles is becoming mainstream.
The selection of refrigeration and freezer units is important and both the EU and US have mandates regarding refrigerants. The Montreal Protocol has banned the use of chlorofluorocarbons and is phasing out the use of hydrochlorofluorocarbons. Some industries can select natural refrigerants such as CO2, or if this is not possible, a refrigerant with a low ozone depletion potential and global warming potential rating. Refrigerant management plans that addresses leak detection or schedule retrofit to phase out older refrigerants are valuable.
Service
Environmental sustainability is promoted by the use of high-efficiency ventilators, air handling units, and chillers, as well as is the use of HVAC units, filters, etc., with reserve power− because greater nominal power consumes more energy. Avoiding unnecessary narrow tolerances for temperature and humidity where feasible is also an advantage.
Facility electrification has several benefits, as described in such international energy management standards as ISO 50001:2018 EnMS,26 European S.I. No. 646 of 2016,27 and the GHG Standard Scope 2.28 Many are now supplanting their energy needs with on-site photoelectric sources on rooftops and carports, and wind turbines. Off-site renewable energy comes from third-party solar power, wind farms, hydroelectric, and geothermal sources. Both are considered better for the environment, but on-site generation is preferred because it increases the generally available supply of renewable energy on the grid.
Advanced energy metering/analysis can identify opportunities to reduce consumption and waste, as well as support sustainability-integrated process control. In an existing building, the first step to reduce energy use is to perform such an energy audit. In a proposed facility, building and process modeling early in the design phase will identify opportunities for improvements and synergies within the systems. An energy modeling system such as the 8760-energy model simulates, hour by hour, the proposed energy usage over a 12-month period.
There is an increase in those issuing a path to net zero design strategy document, which sets out design parameters covering all aspects of a project from initial concept through the commissioning, post-occupancy surveys, and audits. Examples here include using local weather, occupancy loads, and other factors to identify decarbonization strategies, and double commissioning — where the primary evaluation of the facility is followed by a second, post-evaluation and performance data-rich, optimization commissioning.
Geoexchange systems are an application of heat pumps that retrieve and store heat from the earth. The various approaches to these systems, such as ground-source heat pump or geothermal sources, include the popular geothermal piles. According to the EPA, “Geothermal heat pumps can reduce energy consumption and corresponding emissions by up to 44% compared to air-source heat pumps and up to 72% compared to electric resistance heating with standard air-conditioning equipment.”29
Air and liquid heat pumps and exchangers of various design are a means of passive energy recovery/exchange that move heat from one area/material to another. Air/air heat exchangers are commonly employed to supplement system chillers and boilers by conditioning the external air employed in ventilation and can reduce energy demand by up to 90%. External tower air/liquid exchangers produce temperature conditioned fluids from the ambient environment.
The energy efficiency of boilers and furnaces begins with understanding specific volumes, recovery, and temperature requirements. Overall efficiency varies by such engineering as selection of equipment, matching those equipment capacities (average, peak, and recovery), and timing equipment operation to the need.
Daylighting via high-level windows and light shelves re-directing is the most sustainable source, but is sometimes obviated by reasons of layout, security, and product quality. Environmental sustainability is further enabled by automated need-adjustment and the use of LED bulbs decreasing both power demand and thermal load.
Water sourcing, consumption reduction, and reuse is powerful, especially in biopharmaceutical manufacturing. It’s been reported that, on average, 7,711 kg of materials input is required to produce 1 kg of mAb− mostly due to the water-intensive nature of biologics production.30 Reducing water consumption both inside and outside a facility through specific engineering, conversion to SU technologies, and process intensification can reduce not only usage – but the energy required to heat, cool and distribute it. Sub-meters allows the user to understand local usage and support development of future efficiencies.
Beyond demand for the water itself, water for injection (WFI) systems can be a major consumer of energy in biopharmaceutical facilities. Traditional WFI is systems employ distillation, a “hot” process that uses steam. An alternative, energy-efficient “cold” WFI generation utilizing membrane technology has existed for years, but had been seldom implemented due to, e.g., European Pharmacopoeia (EP) requirements. However, as of 2017, the EP revised its guidelines to allow for cold WFI generation.31 It is reported that only about 10% of the operating costs for cold WFI units is energy consumption vs over 50% for hot WFI, and that CO2 emissions are correspondingly 90%-95% lower.32 Cold, ozone-based sanitization cannot completely eliminate the need for high-temperature sanitization but can greatly reduce its required frequency.
The US Energy Policy Act (EPAct) of 1992 established water conservation standards acting as a baseline for water usage in efficient plumbing fixtures. Implementation of aerated faucets, high-efficiency toilets, and waterless urinals results in reductions in water consumption and energy usage and support the recommended 20% indoor water use reduction.33 Fixtures and fittings with a WaterSense label, can achieve a 20% reduction in indoor water use.
Where appropriate, replacing potable with locally sourced, reused, or otherwise nonpotable water is valuable. They can be used for e.g., landscaping irrigation so the demand on municipal water is reduced. For example, a biopharmaceutical manufacturing facility in Puerto Rico uses rainwater and condensation from equipment to cool cleanrooms, and greywater for irrigation purposes. 34
Modern Design Tools
Computer-aided design/building information modeling software and knowledgeable operators are now the only efficient way to balance the many various, sometimes competing, goals in facility design. Modeling capability has evolved to define seven dimensions:
- 3D as geometry (x, y, z)
- 4D as timing and duration
- 5D as cost estimation and budget analysis
- 6D as robustness and environmental sustainability
- 7D as continued facility management
An integrative design team can employ such modeling tools to identify and assess concerns or synergies, indicating opportunities for improvement in e.g., carbon footprint of a building or process.
Building Construction
Building carbon efficiency is determined by such factors as facility footprint, thermal efficiency, volume of air exchange, and geographical setting and evaluated by a formal impact assessment. Green design initiatives — such as the placement of photovoltaic panels on the building envelope — can be powerful in offsetting some of the energy requirements. Passive approaches can also be valuable, such as a green roof, green walls, reflective road and roof surfaces, automated window blinds, and double façade glass.
Through such sustainability-driven choices of building materials as site-sourced recycled concrete, waste disposal and new material production needs are significantly reduced.35 The LEED green building rating system recognizes such approaches in its point system.
Products and systems with an environmental product declaration (EPD) and a health product declaration (HDP) are preferred. Tested and classified cleanroom-suitable materials have been entered into a database belonging to an industrial alliance.36
Building orientation, massing, and fenestration, such as orientating new buildings on a generally east/west axis, where longer elevations face south or north, will most likely give the best energy performance. Building up rather than out has many benefits over the typical sprawling single-story facility.37
Financial Tools
The design team can review which available PACE loans; federal, state, and utility incentives; and foundation grants or low interest loans may be relevant to a particular project.
Conclusion
The biopharmaceutical sector employs a number of certification systems that provide value in various ways, including assisting in the design of an environmental sustainability program. A science-based approach is essential in establishing a comprehensive and efficient sustainability program, and an integrative design process helps identify synergies, costs savings, and economies. Facility design in the biopharmaceutical sector challenges designers to think outside the box and to be innovative in approaches, as it is a dynamic field involving continually evolving goals, metrics, technologies, materials, equipment, service support, and therapeutic modalities.